Structure Optimization and Photothermal Evaporation Performance Evaluation of Graphene/Melamine Sponge
-
摘要: 为解决淡水资源危机,以还原氧化石墨烯(RGO)和三聚氰胺海绵(MS)作为负载材料和基底材料,制备出了蘑菇状结构石墨烯/三聚氰胺海绵复合光热水蒸发材料(MRMS),以减少热量损失,提高蒸发速率。对影响材料结构的因素进行研究,包括总蒸发表面积与投影地面面积的比值(EAI值)、光吸收面积与吸水面积比值(PAI值)及下部输水圆柱的高度等方面。结果显示,高度为7 mm、直径为20 mm的顶部圆柱与高度为12 mm、直径为11 mm的底部输水圆柱组合的MRMS,在一个太阳光条件下蒸发速率最高可达2.41 kg·m−2·h−1,蒸发效率高达91.53%。对最优性能材料进行表征实验、连续使用性能和抗盐性能测定,证明了材料具有稳定的连续使用能力和抗盐性能,在海水淡化方面有良好的应用前景。Abstract: Reduced graphene oxide (RGO) and melamine sponge (MS) were utilized as loading and substrate materials to address the freshwater resource crisis. A mushroom-shaped graphene/melamine sponge composite photothermal water evaporation material (MRMS) was created to increase evaporation rate and decrease heat loss. It was investigated how the material's structure affected how well it performed in photothermal evaporation. The factors investigated include the ratio of total evaporation surface area to projected footprint area (expressed as EAI value), height of the bottom cylinder for water transportation, and the ratio between photothermal area and water absorption area (PAI value). The experimental results indicated that a MRMS formed by a top cylinder, 7 mm in height and 20 mm in diameter, and a bottom water conveying cylinder, 12 mm in height and 11 mm in diameter, showed the best photothermal evaporation performance. Under 1 sun illumination, the evaporation rate reached a maximum value of 2.41 kg·m−2·h−1, with an evaporation efficiency of 91.53%. Characterization experiments on the optimal performance material, along with assessments of its continuous usage performance and salt resistance, demonstrate that the material exhibits stable continuous operational capability and salt resistance, showing application prospect in the field of seawater desalination.
-
Key words:
- solar energy /
- desalination /
- evaporation /
- Graphene /
- Melamine sponge /
- Structural optimization
-
图 3 蘑菇状结构石墨烯/三聚氰胺海绵复合光热水蒸发材料(MRMS)实物图、红外图和不同总蒸发表面积与投影地面面积的比值(EAI值)的MRMS表面温度及蒸发速率变化曲线
Figure 3. Physical image and infrared image of mushroom-shaped graphene/melamine sponge composite photothermal water evaporation material (MRMS) and the variation curves of MRMS surface temperature and evaporation rate with different ratio of total evaporation surface area to projected footprint area (EAI) values
-
[1] SIMONOVIC S P. World water dynamics: global modeling of water resources[J]. Journal of Environmental Management, 2002, 66(3): 249-267. doi: 10.1016/S0301-4797(02)90585-2 [2] 刘强, 肖维新, 罗渊, 等. 高效海水淡化的太阳能界面蒸发器研究进展 [J]太阳能学报. 2024, 45(03): 591-602.LIU Qiang, XIAO Weixin, LUO Yuan, et al. Rsearch progress of solar interface evaporators for high-efficiency desalination[J]. Acta Energiac Solaris Sinica, 2024, 45(03): 591-602(in Chinese). [3] SAAVEDRA A, VALDES H, MAHN A, et al. Comparative Analysis of Conventional and Emerging Technologies for Seawater Desalination: Northern Chile as A Case Study[J]. Membranes, 2021, 11(3): 180. doi: 10.3390/membranes11030180 [4] CHOI S-H. On the brine re-utilization of a multi-stage flashing (MSF) desalination plant[J]. Desalination, 2016, 398: 64-76. doi: 10.1016/j.desal.2016.07.020 [5] BORDBAR B, KHOSRAVI A, AHMADI ORKOMI A, et al. Life Cycle Assessment of Hybrid Nanofiltration Desalination Plants in the Persian Gulf[J]. Membranes, 2022, 12(5): 467. doi: 10.3390/membranes12050467 [6] KALISTA B, SHIN H, CHO J, et al. Current development and future prospect review of freeze desalination[J]. Desalination, 2018, 447: 167-181. doi: 10.1016/j.desal.2018.09.009 [7] GOHIL P P, DESAI H, KUMAI A, et al. Current Status and Advancement in Thermal and Membrane-Based Hybrid Seawater Desalination Technologies[J]. Water, 2023, 15(12): 2274. doi: 10.3390/w15122274 [8] AHMED F E, HASHAIKEH R, HILAL N. Solar powered desalination – Technology, energy and future outlook[J]. Desalination, 2019, 453: 54-76. doi: 10.1016/j.desal.2018.12.002 [9] LIU C, YIN Z, HOU Y, et al. Overview of Solar Steam Devices from Materials and Structures[J]. Polymers, 2023, 15(12): 2742. doi: 10.3390/polym15122742 [10] 罗文琪. 具有多级孔道结构的仿生太阳能光热蒸发器的制备及性能调控 [D], 2022.LUO Wenqi. Preparation and performance modulation of biomimetic solar steam generator with multi-level pore structure[D], 2022(in Chinese). [11] LI Y, SHI Y, WANG H, et al. Recent advances in carbon-based materials for solar-driven interfacial photothermal conversion water evaporation: Assemblies, structures, applications, and prospective[J]. Carbon Energy, 2023, 5(11): e331. doi: 10.1002/cey2.331 [12] ZHU G, JING G, XU G, et al. A green and efficient strategy facilitates continuous solar-induced steam generation based on tea-assisted synthesis of gold nanoflowers[J]. Nano Research, 2022, 15(7): 6705-6712. doi: 10.1007/s12274-022-4269-7 [13] ALSEHLI M. Application of graphene nanoplatelet/platinum hybrid nanofluid in a novel design of solar still for improving water production and energy management[J]. Sustainable Energy Technologies and Assessments, 2022, 53: 102607. doi: 10.1016/j.seta.2022.102607 [14] IBRAHIM I, SEO D H, MCDONAGH A M, et al. Semiconductor photothermal materials enabling efficient solar steam generation toward desalination and wastewater treatment[J]. Desalination, 2021, 500: 114853. doi: 10.1016/j.desal.2020.114853 [15] 吕霈泓, 李娟, 石雷, 等. 基于光热木材的海水淡化研究进展与展望 [J] 太阳能学报. 2024, 45(06): 401-411.LU Peihong, LI Juan, SHI Lei, et al. Research progress and prospect of seawater desalination based on photothermal wood[J]. Acta Energiac Solaris Sinica, 2024, 45(06): 401-411(in Chinese). [16] ZHANG H, LIU H, CHEN S, et al. Preparation of Three-dimensional Graphene-based Sponge as Photo-thermal Conversion Material to Desalinate Seawater[J]. Chemical Research in Chinese Universities, 2022, 38(6): 1425-1434. doi: 10.1007/s40242-022-1500-8 [17] FENG X, SUN D, YANG J. Eco-friendly synthesis of ferric ion-polyphenol-graphene aerogel for solar steam generation[J]. Materials Letters, 2022, 313: 131738. doi: 10.1016/j.matlet.2022.131738 [18] HAN B, ZHANG Y L, CHEN Q D, et al. Carbon-Based Photothermal Actuators[J]. Advanced Functional Materials, 2018, 28(40): 1802235. doi: 10.1002/adfm.201802235 [19] RAZAQ A, BIBI F, ZHENG X, et al. Review on Graphene-, Graphene Oxide-, Reduced Graphene Oxide-Based Flexible Composites: From Fabrication to Applications[J]. Materials, 2022, 15(3): 1012. doi: 10.3390/ma15031012 [20] ZHAO Q, YANG W, ZHANG H, et al. Graphene oxide Pickering phase change material emulsions with high thermal conductivity and photo-thermal performance for thermal energy management[J]. Colloids and Surfaces A: Physicochemical and Engineering Aspects, 2019, 575: 42-49. [21] ZHAO D, FANG Z, TANG Y, et al. Graphene Oxide as an Effective Soil Water Retention Agent Can Confer Drought Stress Tolerance to Paeonia ostii without Toxicity[J]. Environmental Science & Technology, 2020, 54(13): 8269-8279. [22] 苏居文. 海绵负载氧化石墨烯构筑太阳能水蒸发器及其性能的研究 [D], 2022.SU Juwen. Study on solar water evaporator constructed with sponge supported graphene oxide and its properties[D], 2022(in Chinese). [23] CHEN Y, ZHAO X, YE Z, et al. Robust seawater desalination and sewage purification enabled by the solar-thermal conversion of the Janus-type graphene oxide evaporator[J]. Desalination, 2022, 522: 115406. doi: 10.1016/j.desal.2021.115406 [24] YANG Y, LIU C, WANG J, et al. Janus 3D graphene based evaporator with controllable wettability for highly efficient solar desalination[J]. Desalination, 2023, 558: 116639. doi: 10.1016/j.desal.2023.116639 [25] SONG R, ZHANG N, WANG P, et al. A self-floating Janus PPy@Ni sponge salt-resisting solar evaporator for efficient interfacial evaporation[J]. Applied Surface Science, 2023, 616: 156448. doi: 10.1016/j.apsusc.2023.156448 [26] 张德旭. 高速太阳能水蒸发体系的构建及其性能研究 [D], 2021.ZHANG Dexu. Construction and performance of high efficient solar water evaporation system[D], 2021(in Chinese). [27] CHEN X, QU Z, LIU Z, et al. Mechanism of Oxidization of Graphite to Graphene Oxide by the Hummers Method[J]. ACS Omega, 2022, 7(27): 23503-23510. doi: 10.1021/acsomega.2c01963 -
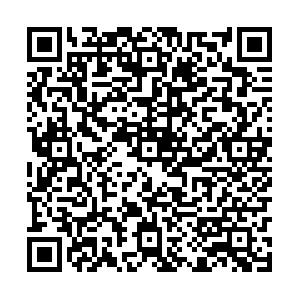
计量
- 文章访问数: 25
- HTML全文浏览量: 22
- 被引次数: 0