Study on ZIF-8@CNTs functionalized stereocomplexed poly(lactic acid) highly electroactive nanofibrous membranes for long-term PMs filtration
-
摘要: 空气污染中的颗粒物(PMs)已成为全球关注的焦点问题。这些微小的颗粒能够携带众多有害物质,并通过呼吸系统进入人体的血液循环,从而对健康造成严重影响,包括呼吸系统和神经系统的疾病。然而,传统的防护口罩过滤性能存在很大的局限性,且不可生物降解的过滤口罩在丢弃后往往会对环境产生负面影响。因此,迫切需要开发一种可降解的过滤纤维膜,其中聚乳酸(PLA)在可生物降解空气过滤材料领域具有巨大潜力。然而,受制于其低电活性特征,使得其在静电吸附颗粒物方面的能力不足,难以达到高效过滤空气颗粒物的目的。本文采用微波辅助法,在碳纳米管(CNTs)上成功合成了ZIF-8纳米晶体,得到表面规整的纳米杂化结构ZIF-8@CNTs。并将左旋聚乳酸(PLLA)和右旋聚乳酸(PDLA)共混,基于静电纺-电喷技术将ZIF-8@CNTs 纳米杂化体嵌入在纤维上得到立构复合化聚乳酸纳米纤维膜(ZIF-8@CNTs/SC-PLA),在纤维表面上形成凸出状结构,增加了纤维的物理拦截以及静电吸附性能。PLLA和PDLA链之间的氢键相互作用可以触发立体复合晶(SCs)的形成,进而影响PLA的结晶度,提高了ZIF-8@CNTs/SC-PLA纳纤膜的极化性能和电活性。通过调整ZIF-8@CNTs的浓度,调控纤维的表面形貌,评估了在不同呼吸状态下所产生的电信号,并探讨了在不同流速下ZIF-8@CNTs/SC-PLA纳纤膜的过滤性能以及压降。结果表明:随着ZIF-8@CNTs浓度的增加,纤维的表面逐渐粗糙,直径呈先减小后增大的趋势,介电常数(2.5)以及表面电势(7.7 kV)得到明显改善。在不同流速下,ZIF-8@CNTs/SC-PLA纤维膜的压降都明显低于Pure PLLA纤维膜,其中,在85 L/min 流速下,10%ZIF-8@CNTs/SC-PLA纳纤膜的压降仅206.9 Pa,这远低于Pure PLLA纤维膜(465.2 Pa),且在32 L/min流速下,10%ZIF-8@CNTs/SC-PLA纳纤膜对PM0.3和PM2.5的过滤效率分别为86.9%和96.1%,相较于Pure PLLA纤维分别提升13.2%和8.6%。且在不同的呼吸状态下10%ZIF-8@CNTs/SC-PLA纳纤膜可实现不同的信号输出,展现出优异的电活性,为自供电便携式呼吸监测防护装备提供了新的设计参考。Abstract: Particulate matter (PMs) in air pollution has become a global concern. These tiny particles are capable of carrying numerous harmful substances and entering the human blood circulation through the respiratory system, resulting in serious health effects, including respiratory and neurological diseases. However, conventional protective masks have significant limitations in filtration performance, and non-biodegradable filter masks often have a negative impact on the environment when discarded. Therefore, there is an urgent need to develop a biodegradable filtration fibrous membrane, in which polylactic acid (PLA) has great potential in the field of biodegradable air filtration materials. However, it is limited by its low electroactivity characteristics, which makes its ability in electrostatic adsorption of particulate matter insufficient to achieve efficient filtration of air particulate matter. In this paper, ZIF-8 nanocrystals were successfully synthesized on carbon nanotubes (CNTs) using a microwave-assisted method to obtain ZIF-8@CNTs nanohybrids with regular surfaces. In addition, poly (L-lactic acid) (PLLA) and poly (D-lactic acid) (PDLA) were blended, and the ZIF-8@CNTs NPs were embedded in the fibers based on electrospinning-electrospray technology to obtain the standing composite poly(lactic acid) fibrous membranes (ZIF-8@CNTs/SC-PLA fibrous membranes), the formation of protruding structures on the fiber surface increases the physical interception as well as the electrostatic adsorption properties of the fibers. The hydrogen bonding interaction between PLLA and PDLA chains can trigger the formation of stereocomplexed crystals (SCs), which in turn affects the crystallinity of PLA and improves the polarization properties and electrical activity of ZIF-8@CNTs/SC-PLA fibrous membranes. By adjusting the concentration of ZIF-8@CNTs and modulating the surface morphology of the fibers, the electrical signals generated under different respiration states were evaluated and the filtration performance as well as the pressure drop of the ZIF-8@CNTs/SC-PLA nanofibrous membranes were explored at different flow rates. The results showed that with the increase of ZIF-8@CNTs concentration, the surface of the fibers was rough, and the diameter of the fibers tended to decrease and then increase, the dielectric constant (2.5) and surface potential (7.7 kV) are significantly improved. The pressure drop of ZIF-8@CNTs/SC-PLA nanofibrous membranes was significantly lower than that of pure PLLA fibrous membrane at different flow rates, among which the pressure drop of 10%ZIF-8@CNTs/SC-PLA nanofibrous membranes was only 206.9 Pa at a flow rate of 85 L/min, which was much lower than that of pure PLLA fibrous membranes (465.2 Pa). And at a flow rate of 32 L/min, the filtration efficiencies of the 10%ZIF-8@CNTs/SC-PLA membrane for PM0.3 and PM2.5 were 86.9% and 96.1%, respectively, which were 13.2% and 8.6% higher than that of the pure PLLA fibrous membranes, respectively. Moreover, the 10%ZIF-8@CNTs/SC-PLA fibrous membranes can achieve different signal outputs under different respiratory states, showing excellent electrical activity, which provides a new design reference for self-powered portable respiratory monitoring and protective equipment.
-
Key words:
- Poly(lactic acid) /
- stereocomplexed /
- electroactivity /
- air filtration /
- respiratory monitoring
-
图 4 不同ZIF-8@CNTs浓度的ZIF-8@CNTs/SC-PLA纳纤膜SEM图 (a) Pure PLLA;(b) 6%ZIF-8@CNTs/SC-PLA;(c) 8%ZIF-8@CNTs/SC-PLA;(d) 10%ZIF-8@CNTs/SC-PLA
Figure 4. SEM image of ZIF-8@CNTs/SC-PLA nanofibrous membranes with different ZIF-8@CNTs mass fractions (a) Pure PLA; (b) 6%ZIF-8@CNTs/SC-PLA; (c) 8%ZIF-8@CNTs/SC-PLA; (d) 10%ZIF-8@CNTs/SC-PLA
图 5 ZIF-8@CNTs/SC-PLA和Pure PLLA纤维直径以及孔隙分布(a) Pure PLLA;(b) 6%ZIF-8@CNTs/SC-PLA;(c) 8%ZIF-8@CNTs/SC-PLA;(d) 10%ZIF-8@CNTs/SC-PLA
Figure 5. ZIF-8@CNTs/SC-PLA and Pure PLLA fiber diameter and pore size distribution (a) Pure PLA; (b) 6%ZIF-8@CNTs/SC-PLA; (c) 8%ZIF-8@CNTs/SC-PLA; (d) 10%ZIF-8@CNTs/SC-PLA
图 10 (a) ZIF-8@CNTs/SC-PLA和Pure PLLA纤维膜的品质因素;(b) 在32 L/min流速下循环测试,10%ZIF-8@CNTs/SC-PLA纳纤维膜对PM0.3和PM2.5的过滤效率;(c) 10%ZIF-8@CNTs/SC-PLA循环过滤后的扫描电镜图
Figure 10. (a) Quality factors of ZIF-8@CNTs/SC-PLA and Pure PLA fibrous membranes; (b) Filtration efficiency of 10%ZIF-8@CNTs/SC-PLA nanofibrous membrane for PM0.3 and PM2.5 tested by cycling at airflow of 32 L/min; (c) SEM image of 10%ZIF-8@CNTs/SC-PLA after cyclic filtration test
-
[1] DOU Y, WANG N, ZHANG S, et al. Electroactive nanofibrous membrane with antibacterial and deodorizing properties for air filtration[J]. Journal of hazardous materials, 2024, 469: 134064. doi: 10.1016/j.jhazmat.2024.134064 [2] 沈峥, 徐超, 张一帆, 等. 高抗湿MOF化聚乳酸纳纤膜制备及其高效滤除PM0.3性能[J]. 复合材料学报. 2024: 1-10.SHEN Zheng, XU Chao, ZHANG Yifan, et al. MOF-functionalized poly(lactic acid) nanofiberous membranes for efficient removal of PM0.3 and increased humidity resistance[J]. Acta Materiae Compositae Sinica. 2024: 1-10 (in Chinese). [3] SUN J, PENG Z, ZHU Z, et al. The atmospheric microplastics deposition contributes to microplastic pollution in urban waters[J]. Water Research, 2022, 225: 119116. doi: 10.1016/j.watres.2022.119116 [4] PIYATHILAKE U, LIN C, BOLAN N, et al. Exploring the hidden environmental pollution of microplastics derived from bioplastics: A review[J]. Chemosphere, 2024, 355: 141773. doi: 10.1016/j.chemosphere.2024.141773 [5] HAN W, RAO D, GAO H, et al. Green-solvent-processable biodegradable poly(lactic acid) nanofibrous membranes with bead-on-string structure for effective air filtration: “Kill two birds with one stone”[J]. Nano Energy, 2022, 97: 107237. doi: 10.1016/j.nanoen.2022.107237 [6] VATANPOUR V, DEHQAN A, PAZIRESH S, et al. Polylactic acid in the fabrication of separation membranes: A review[J]. Separation and Purification Technology, 2022, 296: 121433. doi: 10.1016/j.seppur.2022.121433 [7] YANG Y, ZHONG M, WANG W, et al. Engineering biodegradable bacterial cellulose/polylactic acid multi-scale fibrous membrane via co-electrospinning-electrospray strategy for efficient, wet-stable, durable PM0.3 filtration[J]. Separation and Purification Technology, 2025, 352: 128143. doi: 10.1016/j.seppur.2024.128143 [8] 吕英华, 陈雨阳, 王存民, 等. 基于MOF改性的超细聚乳酸纤维过滤性能强化及其智能监测应用[J]. 复合材料学报. 2024: 1-10.LV Yinghua, CHEN Yuyang, WANG Cunming, et al. Enhanced air filtration performance and intelligent monitoring by MOF functionalized ultrafine poly(lactic acid) fibers[J]. Acta Materiae Compositae Sinica. 2024: 1-10 (in Chinese). [9] ZHAN L, DENG J, KE Q, et al. Grooved Fibers: Preparation Principles Through Electrospinning and Potential Applications[J]. Advanced fiber materials (Online), 2022, 4(2): 203-213. doi: 10.1007/s42765-021-00116-5 [10] SHI S, SI Y, HAN Y, et al. Recent Progress in Protective Membranes Fabricated via Electrospinning: Advanced Materials, Biomimetic Structures, and Functional Applications[J]. Advanced Materials, 2022, 34(17): 07938. [11] SI Y, SHI S, HU J. Applications of electrospinning in human health: From detection, protection, regulation to reconstruction[J]. Nano Today, 2023, 48: 101723. doi: 10.1016/j.nantod.2022.101723 [12] 宋欣译, 唐梦珂, 王存民, 等. 立构复合化聚乳酸纳纤膜的制备及高效滤除PM2.5性能[J]. 高等学校化学学报, 2024, 45(2): 9-16.SONG Xingyi, TANG Mengke, WANG Cunming, et al. Preparation of Stereocomplexed PLA Nanofibrous Membranes with High PM2.5 Filtration Efficiency[J]. Chemical Journal of Chinese Universities, 2024, 45(2): 9-16 (in Chinese). [13] 李小川, 唐梦珂, 朱金佗, 等. 界面立构复合化电活性聚乳酸纳纤膜的制备及高效过滤性能[J]. 高等学校化学学报. 2023, 44(12): 34-42.LI Xiaochuan, TANG Mengke, ZHU Jintuo, et al. Interfacial Stereocomplexation of Electroactive Poly(lactic acid) Nanofibrous Membranes for Efficient Filtration of Airborne PMs[J]. Chemical Journal of Chinese Universities. 2024, 44(12): 34-42 (in Chinese). [14] ZHENG Y, XU S, YU C, et al. Stereocomplexed Materials of Chiral Polymers Tuned by Crystallization: A Case Study on Poly(lactic acid)[J]. Accounts of Materials Research, 2022, 3(12): 1309-1322. doi: 10.1021/accountsmr.2c00208 [15] ZHU G, LI X, LI X, et al. Nanopatterned Electroactive Polylactic Acid Nanofibrous MOFilters for Efficient PM0.3 Filtration and Bacterial Inhibition[J]. ACS Applied Materials & Interfaces, 2023, 15(40): 47145-47157. [16] TANG M, JIANG L, WANG C, et al. Bioelectrets in Electrospun Bimodal Poly(lactic acid) Fibers: Realization of Multiple Mechanisms for Efficient and Long-Term Filtration of Fine PMs[J]. ACS Appl Mater Interfaces, 2023, 15(21): 25919-25931. doi: 10.1021/acsami.3c02365 [17] DENG Y, ZHANG N, HUANG T, et al. Constructing tubular/porous structures toward highly efficient oil/water separation in electrospun stereocomplex polylactide fibers via coaxial electrospinning technology[J]. Applied Surface Science, 2022, 573: 151619. doi: 10.1016/j.apsusc.2021.151619 [18] YADAV A, YADAV P, LABHASETWAR P K, et al. CNT functionalized ZIF-8 impregnated poly(vinylidene fluoride-co-hexafluoropropylene) mixed matrix membranes for antibiotics removal from pharmaceutical industry wastewater by vacuum membrane distillation[J]. Journal of environmental chemical engineering, 2021, 9(6): 106560. doi: 10.1016/j.jece.2021.106560 [19] LI X, ZHU G, TANG M, et al. Biodegradable MOFilters for Effective Air Filtration and Sterilization by Coupling MOF Functionalization and Mechanical Polarization of Fibrous Poly(lactic acid)[J]. ACS Appl Mater Interfaces, 2023, 15(22): 26812-26823. doi: 10.1021/acsami.3c03932 [20] JIANG L, ZHU X, LI J, et al. Electroactive and breathable protective membranes by surface engineering of dielectric nanohybrids at poly(lactic acid) nanofibers with excellent self-sterilization and photothermal properties[J]. Separation and Purification Technology, 2024, 339: 126708. doi: 10.1016/j.seppur.2024.126708 [21] HU Y, KAZEMIAN H, ROHANI S, et al. In situ high pressure study of ZIF-8 by FTIR spectroscopy[J]. Chemical Communications, 2011, 47(47): 12694. doi: 10.1039/c1cc15525c [22] 牛桂玲, 陶银萍, 相恒学, 等. 静电纺纳米纤维膜的制备及其在空气过滤中的应用[J]. 中国材料进展, 2024, 43(6): 513-524. doi: 10.7502/j.issn.1674-3962.202206012NIU Guiling, TAO Yinping, XIANG Hengxue, et al. Preparation of Electrospinning Nanofiber Membranes and Their Application in Air Filtration[J]. Materials China, 2024, 43(6): 513-524 (in Chinese). doi: 10.7502/j.issn.1674-3962.202206012 [23] MIRJALILI M, ZOHOORI S. Review for application of electrospinning and electrospun nanofibers technology in textile industry[J]. Journal of Nanostructure in Chemistry, 2016, 6(3): 207-213. doi: 10.1007/s40097-016-0189-y [24] KE L, YANG T, LIANG C, et al. Electroactive, Antibacterial, and Biodegradable Poly(lactic acid) Nanofibrous Air Filters for Healthcare[J]. ACS Appl Mater Interfaces, 2023, 15(27): 32463-32474. doi: 10.1021/acsami.3c05834 [25] LIU W, SHI Y, SUN Z, et al. Poling-Free Hydroxyapatite/Polylactide Nanogenerator with Improved Piezoelectricity for Energy Harvesting[J]. Micromachines, 2022, 13(6): 889. doi: 10.3390/mi13060889 [26] ZHAO R, CAI S, ZHAO Y, et al. Enhanced stereocomplex crystalline polylactic acids in melt processed enantiomeric bicomponent fiber configurations[J]. International Journal of Biological Macromolecules, 2023, 253: 127123. doi: 10.1016/j.ijbiomac.2023.127123 [27] LI Z, TAN B H, LIN T, et al. Recent advances in stereocomplexation of enantiomeric PLA-based copolymers and applications[J]. Progress in Polymer Science, 2016, 62: 22-72. doi: 10.1016/j.progpolymsci.2016.05.003 [28] MEI L, REN Y, GU Y, et al. Strengthened and Thermally Resistant Poly(lactic acid)-Based Composite Nanofibers Prepared via Easy Stereocomplexation with Antibacterial Effects[J]. ACS Applied Materials & Interfaces, 2018, 10(49): 42992-43002. [29] HUANG W, SHI Y, WANG P, et al. Facile and efficient formation of stereocomplex polylactide fibers drawn at low temperatures[J]. Polymer, 2022, 246: 124743. doi: 10.1016/j.polymer.2022.124743 [30] REN Q, WU M, WENG Z, et al. Promoted formation of stereocomplex in enantiomeric poly(lactic acid)s induced by cellulose nanofibers[J]. Carbohydrate Polymers, 2022, 276: 118800. doi: 10.1016/j.carbpol.2021.118800 [31] QUAN Z, ZU Y, WANG Y, et al. Slip effect based bimodal nanofibrous membrane for high-efficiency and low-resistance air purification[J]. Separation and Purification Technology, 2021, 275: 119258. doi: 10.1016/j.seppur.2021.119258 [32] YANG X, PU Y, LI S, et al. Electrospun Polymer Composite Membrane with Superior Thermal Stability and Excellent Chemical Resistance for High-Efficiency PM2.5 Capture[J]. ACS Appl Mater Interfaces, 2019, 11(46): 43188-43199. doi: 10.1021/acsami.9b15219 [33] FU Q, LIU Y, LIU T, et al. Air-permeable cellulosic triboelectric materials for self-powered healthcare products[J]. Nano Energy, 2022, 102: 107739. doi: 10.1016/j.nanoen.2022.107739 [34] MEENA J S, KHANH T D, JUNG S, et al. Self-Repairing and Energy-Harvesting Triboelectric Sensor for Tracking Limb Motion and Identifying Breathing Patterns[J]. ACS applied materials & interfaces, 2023, 15(24): 29486-29498. -
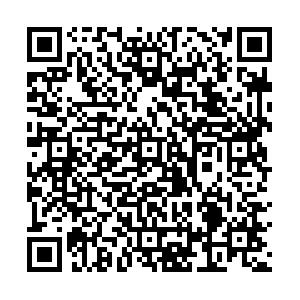
计量
- 文章访问数: 16
- HTML全文浏览量: 12
- 被引次数: 0