A Review of Flexible Thermal Conductive Materials
-
摘要: 随着电子器件向着高集成化、高功率化、一体化和多功能化方向发展,以及可穿戴器件、柔性显示和软体机器人等新型柔性器件的兴起,对器件的高效散热和柔性可变形能力提出了更高的要求,因此柔性散热材料得到越来越多的关注,具有广阔的应用前景。本文综述了柔性散热材料的研究进展和现状,对比分析了碳基类、聚合物类和液态金属类这三大类柔性散热材料的优缺点,指出兼具优良导热性、柔韧性的复合柔性散热材料具有深厚发展潜力和实用价值。Abstract: With the development of electronic devices in the direction of high integration, high power and multifunctionality, as well as the rise of new flexible devices such as wearable devices, flexible displays and soft robots, higher requirements have been put forward for the efficient heat dissipation and flexible deformable ability of the devices, so flexible thermal conductive materials have received more and more attention, and have a broad application prospect. This paper summarizes the research progress and current status of flexible thermal conductive materials, compares and analyzes the advantages and disadvantages of the three major types of flexible thermal conductive materials, namely, carbon-based, polymer-based and liquid metal-based, and points out that the composite flexible thermal conductive materials, which are both excellent in thermal conductivity and flexibility, have profound development potential and practical value.
-
Key words:
- flexible thermal conductive materials /
- thermal interface materials /
- graphene /
- polymer /
- liquid metal
-
图 1 用于个人体温调节的石墨烯基材料:(a)实现均匀有色石墨烯膜的MSAA方法[18]; (b)基于石墨烯纸的剪纸结构可穿戴个人热管理器件[20]; (c) 石墨烯薄膜加热器[19]; (d)共焦显微拉曼光谱测量悬浮单层石墨烯的导热性[11]
Figure 1. Graphene-based materials for personal thermoregulation : (a) The MSAA method to achieve uniform colored graphene film[18]; (b) A paper-cut structured wearable personal thermal management device based on graphene paper[20]; (c) Graphene film heater[19]; (d) Measurement of thermal conductivity of the suspended monolayer graphene by confocal Raman spectroscopy[11]
图 4 掺杂EGaIn的铂催化硅弹性体。(a) 高度变形的LMEE。(比例尺,25 mm)。(b) EGaIn合金在室温下的滴落示意图。(比例尺,10 mm)。(c) LMEE复合材料的示意图。(d)用热风枪加热LMEE和未填充弹性体的交替条带,红外照片时间序列显示LMEE比弹性体更快地散热(图像对应于热源去除后t = 0,5,10和15 s)。(比例尺,25 mm)。[83]
Figure 4. Platinum-catalysed silicone elastomer doped with EGaIn. (a) Highly deformed LMEE. (Scale bar, 25 mm). (b) Schematic illustration of EGaIn alloy droplets at room temperature. (Scale bar, 10 mm). (c) Schematic illustration of LMEE composite material. (d) Alternating strips of LMEE and unfilled elastomer are heated with a heat gun, and the infrared photo sequence shows that LMEE dissipates heat faster than the elastomer (images correspond to t = 0, 5, 10, and 15 s after the heat source is removed). (Scale bar, 25 mm).[83]
表 1 柔性散热材料导热系数对比
Table 1. Comparison of thermal conductivity coefficient of flexible thermal conductive materials
表 2 部分柔性散热材料综合性能对比
Table 2. Comparison of Comprehensive Performance of Partial Flexible Thermal Conductive Materials
材料名称 导热系数W/(m·K) 力学性能描述 应用场景 柔性石墨烯/纤维素纳米纤维复合膜[34] 164.7 在 1000 次弯曲循环后,柔性石墨烯/纤维素纳米纤维复合膜在轴向和径向的热导率几乎没有变化柔性电子设备热管理 3D杂化碳膜[35] 1428 具有43.9 MPa的高抗拉强度和高达5.17 GPa的弹性模量 柔性电子设备热管理 镀镍碳纳米管[36] 1198.5 抗弯强度高达72.3 MPa 5G电子设备热管理 镀铜的聚甲基丙烯酸甲酯[37] 3.38 在30°C时的存储模量高达 1742 MPa高性能电子设备散热 硼氮化物(BN)纳米片/聚二烯丙基二甲基氯化铵复合膜[55] 200 薄膜的杨氏模量和抗拉强度分别为28.3±10.6 MPa和7±0.4 MPa 柔性电子设备以及可穿戴设备散热 双交联水凝胶基纳米复合材料[57] 3.5 具有高达400%的延伸率 小型集成电子设备散热 铂催化硅弹性体中与EGaIn微滴复合材料[82] 9.8 其应变极限超过600% 软体机器人、可穿戴设备散热 -
[1] ZHANG B, WANG L, ZHANG C, et al. , High-performance cellulose nanofiber-derived composite films for efficient thermal management of flexible electronic devices[J]. Chemical Engineering Journal, 2022, 439: 135675. doi: 10.1016/j.cej.2022.135675 [2] HUANG X, GUO W, LIU S, et al. , Flexible mechanical metamaterials enabled electronic skin for real-time detection of unstable grasping in robotic manipulation[J]. Advanced Functional Materials, 2022, 32(23): 2109109. doi: 10.1002/adfm.202109109 [3] 杨斌, 秦文静, 牛永安, 热界面材料发展现状与对策[J]. 科技中国, 2021, (4): 35-37.YANG Bin, QIN Wenjing, NIU Yong'an, Current Status and Countermeasures of Thermal Interface Materials[J]. Science and Technology China, 2021(4): 35-37(in Chinese). [4] 张鑫萍. 双组份液态金属脉动热管传热性能研究[D]. 大连: 大连海事大学, 2020.ZHANG Xinping. Research on Heat Transfer Performance of Two-component Liquid Metal Oscillating Heat Pipe [D]. Dalian: Dalian Maritime University, 2020(in Chinese). [5] MOON S, KIM H, LEE K, et al. , 3D Printable concentrated liquid metal composite with high thermal conductivity[J]. Iscience, 2021, 24: 103183. doi: 10.1016/j.isci.2021.103183 [6] 侯思雨, 闫焕焕, 任芳, 等. 高分子复合材料导热性能的研究进展[J]. 合成材料老化与应用, 2020, 49(6): 135-138.HOU Siyu, YAN Huanhuan, REN Fang, et al. Research Progress on Thermal Conducting Polymer Composites[J]. Synthetic Materials Aging and Application, 2020, 49(6): 135-138(in Chinese). [7] WOOSTER T J, ABROL S, HEY J M, et al. , Thermal, mechanical, and conductivity properties of cyanate ester composites[J]. Composites Part A: Applied science and manufacturing, 2004, 35(1): 75-82. doi: 10.1016/j.compositesa.2003.09.002 [8] NOVOSELOV K S, GEIM A K, MOROZOV S V, et al. Electric field effect in atomically thin carbon films[J]. Science, 2004, 306(5696): 666-669. doi: 10.1126/science.1102896 [9] CAI W, MOORE A L, ZHU Y, et al. Thermal Transport in Suspended and Supported Monolayer Graphene Grown by Chemical Vapor Deposition. [J] Nano Letters, 2010, 10(5) : 1645-1651. [10] FAUGERAS C, FAUGERAS B, ORLITA M, et al. Thermal Conductivity of Graphene in Corbino Membrane Geometry[J]. ACS Nano, 2010, 4(4): 1889-1892. doi: 10.1021/nn9016229 [11] BALANDIN A A, GHOSH S, BAO W, et al. Superior thermal conductivity of single-layer graphene[J]. Nano Letters, 2008, 8(3): 902-907. doi: 10.1021/nl0731872 [12] HU R, LIU Y D, SHIN S M, et al. Emerging Materials and Strategies for Personal Thermal Management[J]. Advanced Energy Materials, 2020, 10(17): 01-23. [13] PARK S, LEE K-S, BOZOKLU G, et al. Graphene oxide papers modified by divalent ions - Enhancing mechanical properties via chemical cross-linking[J]. ACS Nano, 2008, 2(3): 572-578. doi: 10.1021/nn700349a [14] WANG J, LIANG M, FANG Y, et al. Rod-Coating: Towards Large-Area Fabrication of Uniform Reduced Graphene Oxide Films for Flexible Touch Screens[J]. Advanced Materials, 2012, 24(21): 2874-2878. doi: 10.1002/adma.201200055 [15] LI Y, SHENG K, YUAN W, et al. A high-performance flexible fibre-shaped electrochemical capacitor based on electrochemically reduced graphene oxide[J]. Chemical Communication, 2013, 49(3): 291-293. doi: 10.1039/C2CC37396C [16] LI X, CAI W, COLOMBO L, et al. Evolution of Graphene Growth on Ni and Cu by Carbon Isotope Labeling[J]. Nano Letters, 2009, 9(12): 4268-4272. doi: 10.1021/nl902515k [17] XIN G, SUN H, HU T, et al. Large-Area Freestanding Graphene Paper for Superior Thermal Management[J]. Advanced Materials, 2014, 26(26): 4521-4526. doi: 10.1002/adma.201400951 [18] ZHU Z J, ZHANG J, TONG Y L, et al. Reduced Graphene Oxide Membrane Induced Robust Structural Colors toward Personal Thermal Management[J]. Acs Photonics, 2019, 6(1): 116-122. doi: 10.1021/acsphotonics.8b00952 [19] WANG R, XU Z, ZHUANG J, et al. Highly Stretchable Graphene Fibers with Ultrafast Electrothermal Response for Low-Voltage Wearable Heaters[J]. Advanced Electronic Materials, 2017, 3(2): 1-7. [20] GUO Y, DUN C, XU J, et al. Ultrathin, Washable, and Large-Area Graphene Papers for Personal Thermal Management[J]. Small, 2017, 13(44): 1-9. [21] IIJIMA S. Helical microtubules of graphitic carbon[J]. Nature, 1991, 354(6348): 56-58. doi: 10.1038/354056a0 [22] CHE J, CAGIN T, GODDARD W. Thermal Conductivity of Carbon Nanotubes[J]. Nanotechnology, 2000, 11(2): 65-69. doi: 10.1088/0957-4484/11/2/305 [23] ZHANG W, ZHU Z, WANG F, et al. Chirality dependence of the thermal conductivity of carbon nanotubes[J]. Nanotechnology, 2004, 15(8): 936-939. doi: 10.1088/0957-4484/15/8/010 [24] LU L, YI W, ZHANG D L. 3ω method for specific heat and thermal conductivity measurements[J]. Review Scientific Instruments, 2001, 72(7): 2996-3003. doi: 10.1063/1.1378340 [25] QIU L, ZHENG X H, ZHU J, et al. The effect of grain size on the lattice thermal conductivity of an individual polyacrylonitrile-based carbon fiber[J]. Carbon, 2013, 51: 265-273. doi: 10.1016/j.carbon.2012.08.052 [26] KIM P, SHI L, MAJUMDAR A, et al. Thermal Transport Measurements of Individual Multiwalled Nanotubes[J]. Physical Review Letters, 2001, 87(21): 1-4. [27] YU C, SHI L, YAO Z, et al. Thermal Conductance and Thermopower of an Individual Single-Wall Carbon Nanotube[J]. Nano Letters, 2005, 5(9): 1842-1846. doi: 10.1021/nl051044e [28] 王瑾玉, 张永海, 魏进家. 功率器件热界面材料研究进展[J]. 工程热物理学报, 2022, 43(10): 2699-2710.WANG Jinyu, ZHANG Yonghai, WEI Jinjia. A Review of Thermal Interface Materials for Power Devices[J]. Eng Thermophys, 2022, 43(10): 2699-2710(in Chinese). [29] TENG C-C, MA C-C M, CHIOU K-C, et al. , Synergetic effect of thermal conductive properties of epoxy composites containing functionalized multi-walled carbon nanotubes and aluminum nitride[J]. Composites Part B: Engineering, 2012, 43(2): 265-271. doi: 10.1016/j.compositesb.2011.05.027 [30] 陆鹍. 导热型金刚石—环氧树脂复合材料的制备及性能研究[D]. 吉林大学, 2013. [31] WU H, LU C, ZHANG W, et al. Preparation of low-density polyethylene/low-temperature expandable graphite composites with high thermal conductivity by an in situ expansion melt blending process[J]. Materials& Design, 2013, 52(1): 621-629. [32] SHAHIL K M, BALANDIN A A, Graphene–multilayer graphene nanocomposites as highly efficient thermal interface materials[J]. Nano letters, 2012, 12 (2): 861-867. [33] PAN Y, WANG J, WANG N, et al. Effects of Aligned Carbon Nanotube Microcolumns on Mechanical and Thermal Properties of C/SiC Composites Prepared by LA-CVI Methods[J]. J Eur Ceram Soc, 2019, 39(16): 5463-5467. doi: 10.1016/j.jeurceramsoc.2019.08.038 [34] CHEN Y, HOU X, KANG R, et al. Highly flexible biodegradable cellulose nanofiber/graphene heat-spreader films with improved mechanical properties and enhanced thermal conductivity[J]. Journal of Materials Chemistry C, 2018, 6(46): 12739-12745. doi: 10.1039/C8TC04859B [35] LI Y, ZHU Y, JIANG G, et al. Boosting the Heat Dissipation Performance of Graphene/Polyimide Flexible Carbon Film via Enhanced Through-Plane Conductivity of 3D Hybridized Structure[J]. Small, 2020, 16(8): 1-9. [36] LI S, ZHENG Z, LIU S, et al. , Flexible graphite films with high cross-plane thermal conductivity prepared by graphitization of polyimide catalyzed by Ni-coated-CNTs[J]. Journal of Materials Chemistry C, 2023, 11(28): 9527-9538. doi: 10.1039/D3TC01446K [37] MCV A, QVB B, DDNC D, et al. 3D interconnected structure of poly(methyl methacrylate) microbeads coated with copper nanoparticles for highly thermal conductive epoxy composites[J]. Composites Part B-Engineering, 2019, 175(15): 1-8. [38] LEE S H, YU S, SHAHZAD F, et al. Density-tunable lightweight polymer composites with dual-functional ability of efficient EMI shielding and heat dissipation[J]. Nanoscale, 2017, 9(36): 13432-13440. doi: 10.1039/C7NR02618H [39] SIM L C, RAMANAN S, ISMAIL H, et al. , Thermal characterization of Al2O3 and ZnO reinforced silicone rubber as thermal pads for heat dissipation purposes[J]. Thermochimica acta, 2005, 430(1-2): 155-165. doi: 10.1016/j.tca.2004.12.024 [40] YU Y H, MA C, TENG C C, et al. Enhanced thermal and mechanical properties of epoxy composites filled with silver nanowires and nanoparticles[J]. Journal Taiwan Institute of Chemical Engineers, 2013, 44(4): 654-659. doi: 10.1016/j.jtice.2013.01.001 [41] XU J, MUNARI A, DALTON E, et al. Silver nanowire array-polymer composite as thermal interface material[J]. Journal Applied Physics, 2009, 106(12): 1-8. [42] SESHADRI I, ESQUENAZI G L, BORCA-TSSCIUC T, et al. Multifold Increases in Thermal Conductivity of Polymer Nanocomposites through Microwave Welding of Metal Nanowire Fillers[J]. Advanced Materials Interfaces, 2015, 2(15): 1-6. [43] BALACHANDER N, SESHADRI I, MEHTA R J, et al. Nanowire-filled polymer composites with ultrahigh thermal conductivity[J]. Applied Physics Letters, 2013, 102(9): 1-6. [44] BARAKO M T, ROY-PANZER S, ENGLISH T S, et al. Thermal Conduction in Vertically Aligned Copper Nanowire Arrays and Composites[J]. ACS Applied Materials& Interfaces, 2015, 7(34): 19251-19259. [45] BARAKO M T, ISAACSON S G, LIAN F, et al. Dense Vertically Aligned Copper Nanowire Composites as High Performance Thermal Interface Materials[J]. ACS Applied Materials& Interfaces, 2017, 9(48): 42067-42074. [46] WANG S, CHENG Y, WANG R, et al. Highly Thermal Conductive Copper Nanowire Composites with Ultralow Loading: Toward Applications as Thermal Interface Materials[J]. ACS Applied Materials& Interfaces, 2014, 6(9): 6481-6486. [47] BHANUSHALI S, GHOSH P C, SIMON G P, et al. Copper Nanowire-Filled Soft Elastomer Composites for Applications as Thermal Interface Materials[J]. Advanced Materials Interfaces, 2017, 4(17): 1-12. [48] CHEN W, WANG Z, ZHI C, et al. High thermal conductivity and temperature probing of copper nanowire/upconversion nanoparticles/epoxy composite[J]. Composites Science and Technology, 2016, 130(17): 63-69. [49] AHN K, KIM K, KIM J. Thermal conductivity and electric properties of epoxy composites filled with TiO2-coated copper nanowire[J]. Polymer, 2015, 76(1): 313-320. [50] KIM K, AHN K, JU H, et al. Improvement of Insulating and Thermal Properties of SiO2-Coated Copper Nanowire Composites[J]. Industrial Engineering Chemistry Research, 2016, 55(10): 2713-2720. doi: 10.1021/acs.iecr.5b04141 [51] CAO L, ZHU W, LUO B, et al. Construction of Core–Shell Nanowire Arrays in a Cu–Cu2O Film Electrode for High Efficiency in Heat Dissipation[J]. ACS Applied Material Interfaces, 2020, 12(3): 3836-3846. doi: 10.1021/acsami.9b17103 [52] CHIU H-T, SUKACHONMAKLU T, WANG C-H, et al. , Fabrication and characterization of silicon-based ceramic/aluminum nitride as thermally conductive hybrid filler in silicone rubber composite[J]. Materials Chemistry and Physics, 2014, 147(1-2): 11-16. doi: 10.1016/j.matchemphys.2014.05.004 [53] WAN D, WU X, ZHANG W, et al. Thermal conductivity and thermal expansion of few-layer h-BN/Cu composites[J]. Materials Research Bulletin, 2019, 120(1): 1-6. [54] WU K, WANG J, LIU D, et al. Highly Thermoconductive, Thermostable, and Super-Flexible Film by Engineering 1D Rigid Rod-Like Aramid Nanofiber/2D Boron Nitride Nanosheets[J]. Advanced Materials, 2020, 32(8): 1-9. [55] WU Y, XUE Y, QIN S, et al. BN Nanosheet/Polymer Films with Highly Anisotropic Thermal Conductivity for Thermal Management Applications[J]. ACS Applied Materials& Interfaces, 2017, 9(49): 43163-43170. [56] HONG H, JUNG Y H, LEE J S, et al. Anisotropic Thermal Conductive Composite by the Guided Assembly of Boron Nitride Nanosheets for Flexible and Stretchable Electronics[J]. Advanced Functional Materials, 2019, 29(37): 1-10. [57] JIANG H, WANG Z, GENG H, et al. Highly Flexible and Self-Healable Thermal Interface Material Based on Boron Nitride Nanosheets and a Dual Cross-Linked Hydrogel[J]. ACS Applied Materials& Interfaces, 2017, 9(11): 10078-10084. [58] ZHAN K, CHEN Y, XIONG Z, et al. , Low thermal contact resistance boron nitride nanosheets composites enabled by interfacial arc-like phonon bridge[J]. Nature Communications, 2024, 15(1): 2905. doi: 10.1038/s41467-024-47147-1 [59] LI Y, Tian X, YANG W, et al. Dielectric composite reinforced by in-situ growth of carbon nanotubes on boron nitride nanosheets with high thermal conductivity and mechanical strength[J]. Chemical Engineering Journal, 2019, 358(1): 718-724. [60] ZHAO B, WANG S, ZHAO C, et al. Synergism between carbon materials and Ni chains in flexible poly(vinylidene fluoride) composite films with high heat dissipation to improve electromagnetic shielding properties[J]. Carbon, 2018, 127(1): 469-478. [61] Li M, Tang C, Zhang L, et al. A thermally conductive epoxy polymer composites with hybrid fillers of copper nanowires and reduced graphene oxide[J]. Journal Materials Science: Materials in Electronics, 2017, 28(20): 15694-15700. doi: 10.1007/s10854-017-7459-4 [62] ZHANG Z, LI W, Wang X, et al. Low effective content of reduced graphene oxide/silver nanowire hybrids in epoxy composites with enhanced conductive properties[J]. Journal of Materials Science: Materials in Electronics, 2019, 30(8): 7384-7392. doi: 10.1007/s10854-019-01050-4 [63] LI X, ZENG S, E S, et al. Quick Heat Dissipation in Absorption-Dominated Microwave Shielding Properties of Flexible Poly(vinylidene fluoride)/Carbon Nanotube/Co Composite Films with Anisotropy-Shaped Co (Flowers or Chains)[J]. Acs Applied Materials & Interfaces, 2018, 10(47): 40789-40799. [64] KEMALOGLU S, OZKOC G, AYTAC A, Properties of thermally conductive micro and nano size boron nitride reinforced silicon rubber composites[J]. Thermochimica acta, 2010, 499 (1-2): 40-47. [65] ZHANG P, HAO Y, SHI H, et al. , Highly Thermally Conductive and Structurally Ultra-Stable Graphitic Films with Seamless Heterointerfaces for Extreme Thermal Management[J]. Nano-Micro Letters, 2024, 16(1): 58. doi: 10.1007/s40820-023-01277-1 [66] GUO H, ZHAO H, NIU H, et al. , Highly Thermally Conductive 3D Printed Graphene Filled Polymer Composites for Scalable Thermal Management Applications[J]. ACS Nano, 2021, 15(4): 6917-6928. doi: 10.1021/acsnano.0c10768 [67] 位松. 基于液态金属强化传热的双连续相热界面材料研究[D]. 合肥: 中国科学技术大学, 2020.WEI Song. High-performance thermal interface materials with diphase continuous structure reinforced by liquid metal [D]. Hefei: University of Science and Technology of China, 2020(in Chinese). [68] CHEN S, WANG H Z, Zhao R Q, et al. Liquid Metal Composites[J]. Matter, 2020, 2(6): 1446-1480 doi: 10.1016/j.matt.2020.03.016 [69] 周酉林. 基于室温液态金属的可穿戴应力传感器研究[D]. 宁波: 宁波大学, 2018.ZHOU Youlin. Wearable strain sensors based on room-temperature liquid metal [D]. Ningbo: Ningbo University, 2018(in Chinese). [70] 马坤全. 北京: 液态金属芯片散热方法的研究[D]. 中国科学院研究生院(理化技术研究所), 2008.MA Kunqun. Study on Liquid Metal Cooling Method for Thermal Management of Computer Chip [D]. Beijing: Chinese Academy of Sciences (TechnicalInstitute of Physics and Chemistry), 2008(in Chinese). [71] GWINN J P, Webb R L. Performance and tes ting of thermal interface materials[J]. Microelectron J, 2003, 34(3): 215-222. doi: 10.1016/S0026-2692(02)00191-X [72] 王梦婕. 液态金属基和硅橡胶基热界面材料的制备及其导热性能研究[D]. 青岛: 青岛科技大学, 2018.WANG Mengjie. Preparation and thermal conductivity of liquidmetal-based and silicone-based thermal interface materials [D]. Qingdao: Qingdao University of Science and Technology, 2018(in Chinese). [73] 李振明, 刘伟, 赵勇青, 等. 基于液态金属的高热流密度电力设备冷却实验研究[J]. 电工电能新技术, 2017, 36(4): 66-70.LI Zhenming, LIU Wei, ZHAO Yongqing, et al. Experimental study on cooling electric equipment with high heat flux based on liquid metal[J]. Advanced Technology of Electrical Engineering and Energy, 2017, 36(4): 66-70(in Chinese). [74] GAO Y, Liu J. Gallium-based thermal interface material with high compliance and wettability[J]. Applied Physics A: Materials Science and Processing, 2012, 107(3): 701-708. doi: 10.1007/s00339-012-6887-5 [75] YANG E, GUO H, GUO J, et al. Thermal Performance of Low-Melting-Temperature Alloy Thermal Interface Materials[J]. Acta Metallurgica Sinica-english Letters, 2014, 27(2): 290-294. doi: 10.1007/s40195-014-0042-6 [76] HILL R F, Strader J L. Practical utilization of low melting alloy thermal interface materials [C]. in: Twenty-Second Annual IEEE Semiconductor Thermal Measurement And Management Symposium, Dallas: IEEE, 2006.23-27. [77] ROY C K, BHAVNANI S, HAMILTON M C, et al. Investigation into the application of low melting temperature alloys as wet thermal interface materials[J]. International Journal of Heat and Mass Transfer, 2015, 85(1): 996-1002. [78] ROY C K, BHAVNANI S, HAMILTON M C, et al. Accelerated aging and thermal cycling of low melting temperature alloys as wet thermal interface materials[J]. Microelectronics Reliability, 2015, 55(12): 2698-2704. doi: 10.1016/j.microrel.2015.08.020 [79] ROY C K, BHAVNANI S, HAMILTON M C, et al. Durability of Low Melt Alloys as Thermal Interface Materials[J]. Journal of Electronic Packaging, Transactions of the ASME, 2016, 138(1): 1-7. [80] 李根. 液态金属及碳纳米管强化界面传热研究[D]. 大连: 大连海事大学, 2016.LI Gen. Study ofinterface heat transfer enhancement with liquidmetal and carbon nanotube [D]. Dalian: Dalian Maritime University, 2016(in Chinese). [81] SHARMA D, TIWARI R K, SHARMA R, et al. Two-Phase Metallic Thermal Interface Materials Processed Through Liquid Phase Sintering Followed by Accumulative Roll Bonding[J]. IEEE Transactions On Components Packaging And Manufacturing Technology, 2016, 6(1): 58-66. doi: 10.1109/TCPMT.2015.2507175 [82] LIU J, SAHAYM U, DUTTA I, et al. Interfacially engineered liquid-phase-sintered Cu-In composite solders for thermal interface material applications[J]. Journal of Materials Science, 2014, 49(22): 7844-7854. doi: 10.1007/s10853-014-8495-6 [83] BARTLETT M D, KAZEM N, Powell-Palm M J, et al. High thermal conductivity in soft elastomers with elongated liquid metal inclusions[J]. Proceedings of the National Academy of Sciences of the United States of America, 2017, 114(9): 2143-2148. [84] CHU W X, TSENG P H, WANG C C. Utilization of low-melting temperature alloy with confined seal for reducing thermal contact resistance[J]. Applied Thermal Engineering, 2019, 163(1): 1-6. [85] YAN Y, ZHUANG Y, OUYANG H, et al. , Experimental investigation on optimization of the performance of gallium-based liquid metal with high thermal conductivity as thermal interface material for efficient electronic cooling[J]. International Journal of Heat and Mass Transfer, 2024, 226: 125455. doi: 10.1016/j.ijheatmasstransfer.2024.125455 [86] LIU Z, HU Q, GUO S, et al. , Thermoregulating separators based on phase-change materials for safe lithium-ion batteries[J]. Advanced Materials, 2021, 33(15): 2008088. doi: 10.1002/adma.202008088 [87] PU S, FU J, LIAO Y, et al. , Promoting energy efficiency via a self-adaptive evaporative cooling hydrogel[J]. Advanced Materials, 2020, 32(17): 1907307. doi: 10.1002/adma.201907307 [88] SHI Y, WANG C, YIN Y, et al. , Functional soft composites as thermal protecting substrates for wearable electronics[J]. Advanced Functional Materials, 2019, 29(45): 1905470. doi: 10.1002/adfm.201905470 -
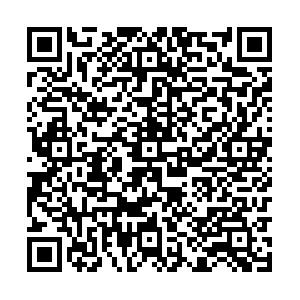
计量
- 文章访问数: 54
- HTML全文浏览量: 29
- 被引次数: 0