Borate modified α-Fe2O3 achieves efficient photocatalytic water splitting for hydrogen production
-
摘要: H2作为一种高效清洁的能源,具有较高的热值(285.8 kJ/mol)、清洁以及来源广等优势,是最有希望替代化石燃料的能源之一。在全球低碳能源政策的推动下,通过光电化学池进行光电催化分解水成为一种前景广阔且环保可持续的制氢方法,而制备廉价且稳定的半导体催化剂是其中的关键步骤。α-Fe2O3作为光阳极具有高理论光电转化效率和稳定性,但是也存在导电性差,空穴扩散长度和激子较短等弊端。为了提高其光电性能,通过浸渍-退火方法在α-Fe2O3纳米棒阵列表面负载了一层超薄的无定型硼酸盐助催化剂(BC)纳米层,制备了BC/α-Fe2O3光阳极催化剂用于光电催化分解水制氢。随后,本文分析了该光阳极催化剂的成分组成、形貌结构、表面状态、光谱吸收特性及分子价态形式,测试并对比了不同BC的制备和负载条件对光电性能的影响,研究了BC在光电催化分解水过程中的作用机制。研究结果显示,BC的负载显著降低了α-Fe2O3纳米棒阵列表面的陷阱密度和析氧过电位,提高了电荷分离效率,从而增强了光阳极的光电催化活性。在100 mW/cm2光照强度和0.1 mol/L KOH电解质中,1.23 V(相对于可逆氢电极)外加偏压的条件下,BC/α-Fe2O3光阳极获得了1.50 mA/cm2的光电流密度,单色光转化效率达到了26%(λ=360 nm)。Abstract: H2, as an efficient and clean energy source, possesses advantages such as high calorific value (285.8 kJ/mol), cleanliness, and wide availability, making it one of the most promising alternatives to fossil fuels. Under the global push for low-carbon energy policies, photoelectrocatalytic water splitting using photoelectrochemical cells has emerged as a promising and environmentally sustainable method for hydrogen production. A critical step in this process is the preparation of cost-effective and stable semiconductor catalysts. As a photoanode, α-Fe2O3 has high theoretical photoelectric conversion efficiency and stability, but it also has drawbacks such as poor conductivity, short hole diffusion length and excitons. In order to improve its photoelectric performance, an ultrathin amorphous borate cocatalyst (BC) layer was deposited on the surface of the α-Fe2O3 nanorod array using a dip-coating and annealing method, resulting in the BC/α-Fe2O3 photoanode catalyst for photoelectrocatalytic water splitting to produce hydrogen. Subsequently, the composition, morphology, surface state, spectral absorption characteristics, and molecular valence forms of the photoanode catalyst were analyzed. The impact of different preparation and loading conditions of the BC on the photoelectric performance was tested and compared, and the role of BC in the photoelectrocatalytic water splitting process was investigated. The research results indicated that the loading of the BC significantly reduced the trap density and oxygen evolution overpotential on the surface of the α-Fe2O3 nanorod array, thereby improving charge separation efficiency and enhancing the photoelectrocatalytic activity of the photoanode. Under conditions of 100 mW/cm2 light intensity, 0.1 mol/L KOH solution, and an applied bias of 1.23 V (relative to the reversible hydrogen electrode), the BC/α-Fe2O3 photoanode achieves a photocurrent density of 1.50 mW/cm2, with a monochromatic light conversion efficiency of 26% (λ=360 nm).
-
Key words:
- photoelectrochemical cell /
- photoelectrocatalysis /
- water splitting /
- hematite /
- surface modification
-
图 2 (a,d) α-Fe2O3、(b,e) BC/α-Fe2O3和(c,f) α-Fe2O3-B光阳极的俯视图和截面图;BC/α-Fe2O3光阳极的(g) EDS光谱和元素映射图、(h) TEM以及(i)高分辨TEM图
Figure 2. Top view and cross-sectional view of (a, d) α-Fe2O3, (b, e) BC/α-Fe2O3, and (c, f) α-Fe2O3-B photoanodes; (g) EDS spectrum and elemental mapping images, (h) TEM and (i) high-resolution TEM images of the BC/α-Fe2O3 photoanode
图 5 AM 1.5 G和0.1 mol/L KOH条件下,(a)不同浸渍时间和(b)退火温度的BC/α-Fe2O3光阳极LSV曲线,在最优条件下BC/α-Fe2O3,α-Fe2O3-B和α-Fe2O3光阳极(c) LSV、(d) IPCE、(e) ABPE和(d) M-S曲线
Figure 5. Under AM 1.5 G and 0.1 mol/L KOH conditions, (a) LSV curves of BC/α-Fe2O3photoanodes with different immersion times, and (b) LSV curves with varying annealing temperatures. Under optimal conditions, (c) LSV, (d) IPCE, (e) ABPE, and (f) M-S plots for BC/α-Fe2O3, α-Fe2O3-B, and α-Fe2O3 photoanodes
图 6 AM 1.5 G,BC/α-Fe2O3,α-Fe2O3-B和α-Fe2O3光阳极在0.1 mol/L KOH中的(a) OCP曲线、(b) OCP数据图、(c) EIS曲线和拟合电路图,以及在0.1 mol/L KOH(0.5 mol/L Na2SO3)测试的(d) LSV、(e)、ηbulk和(f)ηsurface曲线
Figure 6. Under AM 1.5 G, (a) OCP curves, (b) OCP data plots, (c) EIS curves and fitted circuit diagrams of BC/α-Fe2O3, α-Fe2O3-B, and α-Fe2O3 photoanodes in 0.1 mol/L KOH, and (d) LSV, (e) ηbulk, and (f) ηsurface plots tested in 0.1 mol/L KOH (0.5 mol/L Na2SO3)
表 1 α-Fe2O3基光阳极性质参数对比表
Table 1. Comparison of property parameters of α-Fe2O3-based photoanodes
Photoanode Eg/eV Current Density/(mW·cm2) IPCE ABPE Nd/cmbx53 Vfb/mV OCP/VRHE Rct,bulk/Ω α-Fe2O3 2.03 0.62,1.23 VRHE 15.5% 0.080% 7.1×1019 823.9 0.12 988.9 α-Fe2O3-B 2.01 0.98,1.23 VRHE 24.6% 0.120% 7.4×1019 962.5 0.15 531.9 BC/α-Fe2O3 2.01 1.50,1.23 VRHE 26.0% 0.145% 7.9×1019 388.6 0.23 323.1 Notes: Eg (Band gap); IPCE (Incident photon-to-electron conversion efficiency); ABPE (Applied potential photoconversion efficiency); Nd (Donor concentration); Vfb (Flat band potential); OCP (Open-circuit voltage); Rct (Bulk charge transfer resistance). 表 2 BC/α-Fe2O3光阳极与其它材料的性质参数对比
Table 2. Comparison of property parameters between BC/α-Fe2O3 photoanode and other materials
Photoanode Electrolyte Current density/(mW·cm2) IPCE Reference α-Fe2O3@CoBi 0.1 mol/L NaBi 1.12,1.23 VRHE 32.0% [46] α-Fe2O3-B/Ti 1 mol/L NaOH 1.92,1.23 VRHE 35.0% [45] α-Fe2O3@NiFeP 0.1 mol/L KOH 1.75,1.23 VRHE 46.6% [55] α-Fe2O3@Ru 0.1 mol/L KOH 1.42,1.23 VRHE — [53] α-Fe2O3-Sn/NiFeOx 1 mol/L KOH 2.10,1.23 VRHE 13.0% [44] α-Fe2O3/NiFeOOH 1.0 mol/L Na2SO4 1.83,1.23 VRHE 41.0% [42] BC/α-Fe2O3 0.1 mol/L KOH 1.50,1.23 VRHE 26.0% 本文 -
[1] LE T T, SHARMA P, BORA B J, et al. Fueling the future: A comprehensive review of hydrogen energy systems and their challenges[J]. International Journal of Hydrogen Energy, 2024, 54: 791-816. doi: 10.1016/j.ijhydene.2023.08.044 [2] DA SILVA VERAS T, MOZER T S, DA COSTA RUBIM MESSEDER DOS SANTOS D, et al. Hydrogen: Trends, production and characterization of the main process worldwide[J]. International Journal of Hydrogen Energy, 2017, 42(4): 2018-2033. doi: 10.1016/j.ijhydene.2016.08.219 [3] 李仲明, 李斌, 冯东, 等. 锂离子电池正极材料研究进展[J]. 复合材料学报, 2022, 39(2): 513-527.LI Z, LI B, FENG D, et al. Research progress of cathode materials for lithium-ion battery[J]. Acta Materiae Compositae Sinica, 2022, 39(2): 513-527(in Chinese). [4] 付楚芮. 煤炭行业甲烷排放的影响因素与利用途径[J]. 煤质技术, 2024, 39(4): 40-47.FU C. Influencing factors and utilization pathways of methane emission in coal industry[J]. Coal Quality Technology, 2024, 39(4): 40-47(in Chinese). [5] 赵勇, 刘敏, 阙建磊, 等. 煤基低维碳材料制备及其在有机太阳能电池的应用[J]. 煤炭科学技术, 2024, https://link.cnki.net/urlid/11.2402.TD.20240906.0939.001.ZHAO Y, LIU M, QUE J, et al. Preparation of coal-based low-dimension carbon materials and the applications in organic solar cells[J]. Coal Science and Technology, 2024, https://link.cnki. net/urlid/11.2402.TD.20240906.0939.001 (in Chinese). [6] PANCHENKO V A, DAUS Y V, KOVALEV A A, et al. Prospects for the production of green hydrogen: Review of countries with high potential[J]. International Journal of Hydrogen Energy, 2023, 48(12): 4551-4571. doi: 10.1016/j.ijhydene.2022.10.084 [7] 何雅玲, 李印实. 氢能技术科技前沿与挑战[J]. 科学通报, 2022, 67(19): 2113-2114. doi: 10.1360/TB-2022-0648HE Y, LI Y. Frontiers and challenges in hydrogen energy[J]. Chinese Science Bulletin, 2022, 67(19): 2113-2114(in Chinese). doi: 10.1360/TB-2022-0648 [8] 王朔, 张军. 我国制氢技术专利发展与工艺路线研究[J]. 储能科学与技术, 2018, 7(2): 354-362.WANG S, ZHANG J. Research on patent status and process route of hydrogen production in China[J]. Energy Storage Science and Technology, 2018, 7(2): 354-362(in Chinese). [9] ZHAO X, MA X, CHEN B, et al. Challenges toward carbon neutrality in China: Strategies and countermeasures[J]. Resources, Conservation and Recycling, 2022, 176: 105959. doi: 10.1016/j.resconrec.2021.105959 [10] ZHAO Y, LIU X, DING M, et al. Decreasing the molecular polarity of naphthalimide by a π-bridge strategy as cathode interlayers to enable high-efficient electron transfer in organic solar cells[J]. ACS Applied Energy Materials, 2024, 7(7): 2633-2641. doi: 10.1021/acsaem.3c02945 [11] 龙腾文, 王智皓, 何颖超, 等. 光催化燃料电池中污染物净化与重金属还原的协同作用[J]. 聊城大学学报, 2023, 36(6): 45-56.LONG T, WANG Z, HE Y, et al. Synergistic effects of pollutant purificationand heavy Mmetal reductionin photocatalytic fuel cells[J]. Journal of Liaocheng University (Nat. Sci.), 2023, 36(6): 45-56(in Chinese). [12] 王智皓, 龙腾文, 冯建光, 等. SiC量子点敏化g-C3N4极在光催化燃料电池中的应用[J]. 聊城大学学报, 2024, 37(4): 78-88.WANG Z, LONG T, FENG J, et al. Application of SiC quantum dot sensitized g-C3N4 photoanode in photocatalytic fuel cell[J]. Journal of Liaocheng University (Nat.Sci.), 2024, 37(4): 78-88(in Chinese). [13] 曹邵文, 周国庆, 蔡琦琳, 等. 太阳能电池综述: 材料、政策驱动机制及应用前景[J]. 复合材料学报, 2022, 39(5): 1847-1858.CAO S, ZHOU G, CAI Q, et al. A review of solar cells: Materials, policy-driven mechanisms and application prospects[J]. Acta Materiae Compositae Sinica, 2022, 39(5): 1847-1858(in Chinese). [14] FUJISHIMA A, HONDA K. Electrochemical photolysis of water at a semiconductor electrode[J]. Nature, 1972, 238(5358): 37-38. doi: 10.1038/238037a0 [15] 符淑瑢, 王丽娜, 王东伟, 等. 析氧助催化剂增强光阳极光电催化分解水性能研究进展[J]. 化工进展, 2023, 42(5): 2353-2370.FU S, WANG L, WANG D, et al. Oxygen evolution cocatalyst enhancing the photoanode performances for photoelectrochemical water splitting[J]. Chemical Industry and Engineering Progress, 2023, 42(5): 2353-2370(in Chinese). [16] WALTER M G, WARREN E L, MCKONE J R, et al. Solar water splitting cells[J]. Chemical Reviews, 2010, 110: 6446-6473. doi: 10.1021/cr1002326 [17] SURYAWANSHI M P, GHORPADE U V, TOE C Y, et al. Earth-abundant photoelectrodes for water splitting and alternate oxidation reactions: Recent advances and future perspectives[J]. Progress in Materials Science, 2023, 134: 101073. doi: 10.1016/j.pmatsci.2023.101073 [18] LI Z, FANG S, SUN H, et al. Solar hydrogen[J]. Advanced Energy Materials, 2023, 13(8): 2203019. doi: 10.1002/aenm.202203019 [19] 王开放, 刘光, 高旭升, 等. α-Fe2O3光电催化分解水制备氢气研究进展[J]. 化工进展, 2017, 36(2): 397-409.WANG K, LIU G, GAO X, et al. Hematite photoanodes for solar water splitting[J]. Chemical Industry and Engineering Progress, 2017, 36(2): 397-409(in Chinese). [20] LIU B, WANG X, ZHANG Y, et al. A BiVO4 photoanode with a VOx layer bearing oxygen vacancies offers improved charge transfer and oxygen evolution kinetics in photoelectrochemical water splitting[J]. Angewandte Chemie International Edition, 2023, 62(10): e202217346. doi: 10.1002/anie.202217346 [21] FU S, LEWIS D, VAN EYK P, et al. Theoretical screening of single atom doping on β-Ga2O3 (100) for photoelectrochemical water splitting with high activity and low limiting potential[J]. Nanoscale, 2023, 15(15): 6913-6919. doi: 10.1039/D3NR00149K [22] SHEN R, LIANG G, HAO L, et al. In situ synthesis of chemically bonded 2D/2D covalent organic frameworks/O-vacancy WO3 Z-scheme heterostructure for photocatalytic overall water splitting[J]. Advanced Materials, 2023, 35(33): 2303649. doi: 10.1002/adma.202303649 [23] ZHANG W, HOU J , BAI M, et al. Construction of novel ZnO/Ga2SSe (GaSe) vdW heterostructures as efficient catalysts for water splitting[J]. Applied Surface Science, 2023, 634: 157648. [24] QUTB S R, TAWFIK W Z, EL-DEK S I, et al. Superior photoelectrodes of nanostructured Mo-doped CuO thin film for green hydrogen generation from photoelectrochemical water-splitting[J]. International Journal of Hydrogen Energy, 2024, 76: 190-201. doi: 10.1016/j.ijhydene.2024.03.200 [25] Kevin S, Florian L F, Michael G. Solar water splitting: Progress using hematite (α-Fe2O3) photoelectrodes[J]. ChemSusChem, 2011, 4(4): 432-449. doi: 10.1002/cssc.201000416 [26] SHARMA P, JANG J W, LEE J S. Key strategies to advance the photoelectrochemical water splitting performance of α-Fe2O3 photoanode[J]. ChemCatChem, 2018, 11(1): 157-179. [27] GAO R-T, ZHANG J, HE J, et al. Single-atomic-site platinum steers photogenerated charge carrier lifetime of hematite nanoflakes for photoelectrochemical water splitting[J]. Nature Communications, 2023, 14(1): 2640. doi: 10.1038/s41467-023-38343-6 [28] YANG W, PRABHAKAR R R, TAN J, et al. Strategies for enhancing the photocurrent, photovoltage, and stability of photoelectrodes for photoelectrochemical water splitting[J]. Chemical Society Reviews, 2019, 48(19): 4979-5015. doi: 10.1039/C8CS00997J [29] LU C, ZHANG D, WU Z, et al. Hetero phase modulated hematite photoanodes for practical solar water splitting[J]. Applied Catalysis B: Environmental, 2023, 331: 122695. doi: 10.1016/j.apcatb.2023.122695 [30] PARK J, KANG J, CHAULE S, et al. Recent progress and perspectives on heteroatom doping of hematite photoanodes for photoelectrochemical water splitting[J]. Journal of Materials Chemistry A, 2023, 11(45): 24551-24565. doi: 10.1039/D3TA04520J [31] KUDO A, MISEKI Y. Heterogeneous photocatalyst materials for water splitting[J]. Chemical Society Reviews, 2009, 38(1): 253-278. doi: 10.1039/B800489G [32] NICLAS B, LIONEL V, LINDQUIST S-E, et al. Photoelectrochemical studies of oriented nanorod thin films of hematite[J]. Journal of The Electrochemical Society, 2000, 147(7): 2456. doi: 10.1149/1.1393553 [33] KAY C, ANDREAS K, JOSE A, et al. Translucent thin film Fe2O3 photoanodes for efficient water splitting by sunlight: Nanostructure-directing effect of Si-doping[J]. Journal of the American Chemical Society, 2006, 128: 4582-4583. doi: 10.1021/ja060292p [34] LIU H, FAN X, LI Y, et al. Hematite-based photoanodes for photoelectrochemical water splitting: Performance, understanding, and possibilities[J]. Journal of Environmental Chemical Engineering, 2023, 11(1): 109224. doi: 10.1016/j.jece.2022.109224 [35] ZHOU D, FAN K. Recent strategies to enhance the efficiency of hematite photoanodes in photoelectrochemical water splitting[J]. Chinese Journal of Catalysis, 2021, 42(6): 904-919. doi: 10.1016/S1872-2067(20)63712-3 [36] WANG S, CHEN P, BAI Y, et al. New BiVO4 dual photoanodes with enriched oxygen vacancies for efficient solar-driven water splitting[J]. Advanced Materials, 2018, 30(20): 1800486. doi: 10.1002/adma.201800486 [37] YE S, SHI W, LIU Y, et al. Unassisted photoelectrochemical cell with multimediator modulation for solar water splitting exceeding 4% solar-to-hydrogen efficiency[J]. Journal of the American Chemical Society, 2021, 143(32): 12499-12508. doi: 10.1021/jacs.1c00802 [38] JUN S E, HONG S P, CHOI S, et al. Boosting unassisted alkaline solar water splitting using silicon photocathode with TiO2 nanorods decorated by edge-rich MoS2 nanoplates[J]. Small, 2021, 17(39): 2103457. doi: 10.1002/smll.202103457 [39] ZHONG D. K. , SUN J, HIROKI I, et al. Solar water oxidation by composite catalyst/r-Fe2O3 photoanodes[J]. Journal of the American Chemical Society, 2009, 131(17): 6086-6087. [40] TILLEY S D, CORNUZ M, SIVULA K, et al. Light-induced water splitting with hematite: Improved nanostructure and iridium oxide catalysis[J]. Angewandte Chemie International Edition, 2010, 49(36): 6405-6408. doi: 10.1002/anie.201003110 [41] FEHR A M K, AGRAWAL A, MANDANI F, et al. Integrated halide perovskite photoelectrochemical cells with solar-driven water-splitting efficiency of 20.8%[J]. Nature Communications, 2023, 14(1): 3797. doi: 10.1038/s41467-023-39290-y [42] CHEN D, LIU Z, ZHANG S. Enhanced PEC performance of hematite photoanode coupled with bimetallic oxyhydroxide NiFeOOH through a simple electroless method[J]. Applied Catalysis B: Environmental, 2020, 265: 118580. doi: 10.1016/j.apcatb.2019.118580 [43] ZHANG J, Rodrigo G-R, Petra C, et al. Role of cobalt-iron (oxy) hydroxide (CoFeOx) as oxygen evolution catalyst on hematite photoanodes[J]. Energy & Environmental Science, 2018, 11(10): 2972-2984. [44] SEENIVASAN S, ADHIKARI S, KIM D-H. Surface restructuring of hematite photoanodes through ultrathin NiFeOx Catalyst: Amplified charge collection for solar water splitting and pollutant degradation[J]. Chemical Engineering Journal, 2021, 422: 130137. doi: 10.1016/j.cej.2021.130137 [45] AHN H-J, YOON K-Y, KWAK M-J, et al. Boron doping of metal-doped hematite for reduced surface recombination in water splitting[J]. ACS Catalysis, 2018, 8(12): 11932-11939. doi: 10.1021/acscatal.8b03184 [46] XI L, SCHWANKE C, ZHOU D, et al. In situ XAS study of CoBi modified hematite photoanodes[J]. Dalton Trans, 2017, 46(45): 15719-15726. doi: 10.1039/C7DT02647A [47] CAO Y, YIN X, GAN Y, et al. Coupling effect and electronic modulation for synergistically enhanced overall alkaline water splitting on bifunctional Fe-doped CoBi/CoP nanoneedle arrays[J]. Journal of Colloid and Interface Science, 2023, 652: 1703-1711. doi: 10.1016/j.jcis.2023.08.175 [48] FANG W, ZHU Z, YU J, et al. First principles study on high-efficient overall water splitting by anchoring cobalt boride with transition metal atoms[J]. International Journal of Hydrogen Energy, 2024, 53: 1310-1322. doi: 10.1016/j.ijhydene.2023.11.347 [49] EHSAN M A, ULLAH Z, NAZAR M F, et al. Rapid synthesis of nickel-iron-oxide (NiFeOx) solid-solution nano-rods thin films on nickel foam as advanced electrocatalyst for sustained water oxidation[J]. Materials Today Sustainability, 2023, 23: 100451. doi: 10.1016/j.mtsust.2023.100451 [50] LUO Z, LI C, LIU S, et al. Gradient doping of phosphorus in Fe2O3 nanoarray photoanodes for enhanced charge separation[J]. Chemical Science, 2017, 8(1): 91-100. doi: 10.1039/C6SC03707K [51] MAYER M T, DU C, WANG D. Hematite/Si nanowire dual-absorber system for photoelectrochemical water splitting at low applied potentials[J]. Journal of the American Chemical Society, 2012, 134(30): 12406-12409. doi: 10.1021/ja3051734 [52] GUO Q, ZHAO Q, CRESPO-OTERO R, et al. Single-atom iridium on hematite photoanodes for solar water splitting: catalyst or spectator?[J]. Journal of the American Chemical Society, 2023, 145(3): 1686-1695. doi: 10.1021/jacs.2c09974 [53] LI T-T, CUI J-Y, XU M, et al. Efficient acidic photoelectrochemical water splitting enabled by Ru single atoms anchored on hematite photoanodes[J]. Nano Letters, 2024, 24(3): 958-965. doi: 10.1021/acs.nanolett.3c04374 [54] THOMAZ K T C, BEDIN K C, RODRíGUEZ-GUTIéRREZ I, et al. Interfacial engineering of hematite photoanodes toward high water splitting performance[J]. Materials Today Energy, 2023, 37: 101399. doi: 10.1016/j.mtener.2023.101399 [55] LIU G, ZHAO Y, YAO R, et al. Realizing high performance solar water oxidation for Ti-doped hematite nanoarrays by synergistic decoration with ultrathin cobalt-iron phosphate nanolayers[J]. Chemical Engineering Journal, 2019, 355: 49-57. doi: 10.1016/j.cej.2018.08.100 [56] LI Y, ZHANG N, LIU C, et al. Metastable-phase β-Fe2O3 photoanodes for solar water splitting with durability exceeding 100 h[J]. Chinese Journal of Catalysis, 2021, 42: 1992-1998. doi: 10.1016/S1872-2067(21)63822-6 [57] HU Y, AN L, HAN X, et al. Preparation of RhO2 modified BiVO4 thin film photoanodes and their photoelectrocatalytic water splitting performance[J]. Journal of Inorganic Materials, 2022, 37(8): 873-882. -
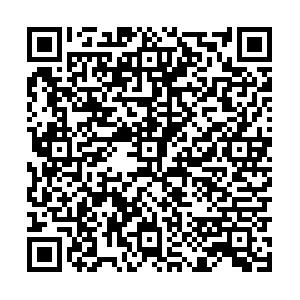
计量
- 文章访问数: 23
- HTML全文浏览量: 17
- 被引次数: 0