Research progress of waterborne polyurethane-based flexible sensing materials
-
摘要: 近年来,柔性传感器在可穿戴电子设备、医疗保健设备、电子皮肤和软体机器人等方面具有广泛的应用前景。聚氨酯(PU)是一类应用于柔性传感领域的基础材料,具有类弹性体、柔韧性及相容性等优势,能满足人们对柔性传感器更好的拉伸性、更高的灵敏度和更宽的工作范围的需求。但随着环保意识的增强,水性聚氨酯(WPU)凭借环保性、低挥发性、易处理性等特性,成为柔性传感领域的研究热点。本文对WPU基柔性传感材料的最新进展进行了跟踪和讨论,包括WPU合成、柔性传感原理、导电填料种类等。最后总结了WPU基柔性传感器独特的多功能性及其广泛应用,并对WPU基柔性传感材料的未来发展前景进行了展望。Abstract: In recent years, flexible sensors have a wide range of applications in wearable electronic devices, healthcare devices, electronic skin and soft robots. Polyurethane (PU) is a class of basic materials used in the field of flexible sensing, with the advantages of elastomer-like, flexibility and compatibility, which can meet the demand for better stretchability, higher sensitivity and wider working range of flexible sensors. However, with the increasing awareness of environmental protection, waterborne polyurethane (WPU) has become a research hotspot in the field of flexible sensing by virtue of its environmental friendliness, low volatility, and ease of handling. In this paper, the recent progress of WPU-based flexible sensing materials is tracked and discussed, including WPU synthesis, flexible sensing principles, and conductive filler types. Finally, the unique multifunctionality of WPU-based flexible sensors and their wide range of applications are summarized, and the future development prospects of WPU-based flexible sensing materials are outlooked.
-
图 3 (a) 传感材料的制备过程[36], (b)复合材料的SEM图[36], (c)g-MWCNTs复合材料的制备过程[37], (d)聚合物中均匀分布的g-MWCNT的扫描电镜图[37]
Figure 3. (a) Fabrication process of the sensor[36], (b) SEM image of the composite material[36], (c) Fabrication process of g-MWCNTs composite[37], (d) SEM of the g-MWCNTs distributed in the polymer uniformly[37]
图 4 (a) 红外光谱和核磁共振谱[39], (b)复合材料FGMWPU的制备过程[39], (c)气凝胶的SEM[42], (d)图实时监测各种人体的活动[42], (e)对身体各部位的实时监测[44]
Figure 4. (a) FTIR spectra and 1H NMR spectra[39], (b) Preparation of FGMWPU composite materials[39], (c) SEM image of aerogel[42], (d) Real-time monitoring of various human body activities[42], (e) Real-time monitoring of all body parts[44]
图 5 (a) 由链缠结和多重氢键构建的物理交联网络[46], (b) 不同温度下材料的愈合效果[46], (c)TCNC的SEM和TEM图像[48], (d) 不同TCNC比例的WPU薄膜的代表性应力-应变曲线和力学数据[48]
Figure 5. (a) Physical cross-linking networks constructed by chain entanglements and multiple hydrogen bonds, (b) Healing effect of materials at different temperatures[46], (c) SEM and TEM images[48], (d) Representative stress-strain curves and mechanical data of WPU films with different ratio of TCNCs[48]
图 6 (a) 聚苯胺接枝到WPU表面的过程[52], (b) 传感器结构及工作原理[52], (c) PEDOT:PSS和WPU的化学结构以及生产PEDOT:PSS/WPU复合纤维的湿法纺丝装置示意图[53], (d) 拉伸应变曲线及循环曲线[53]
Figure 6. (a) the process of grafting polyaniline on the WPU surface[52], (b) Sensor structure and working principle [52], (c) Chemical structures of PEDOT:PSS and WPU and schematic illustration of the wet-spinning set-up for producing PEDOT:PSS/WPU composite fibers[53], (d) Tensile Strain Curve and Cycle Curve[53]
图 7 (a) Ti3C2-MXene 纳米片的制备[55], (b) 纯Ti3C2-MXene 沉积层和Ti3C2/CNCs复合沉积层的断裂机制[55], (c)复合材料制备过程[56], (d) 银纳米线和MXene的分布示意图[56]
Figure 7. (a) Preparation of Ti3C2-MXene Nanosheets[55], (b)Fracture mechanism of pure Ti3C2-MXene deposition layer and Ti3C2/CNCs composite deposition layer[55], (c) Composite material preparation process[56], (d) Schematic distribution of silver nanowires and MXene[56]
图 8 (a)实时监测身体部位和响应速度[48], (b) 由蓝牙模块和智能手机组装而成的人体运动传感系统示意图[62], (c) 复合材料在电子皮肤上的应用[59],(d)长时间下与皮肤的黏附测试[60]
Figure 8. (a) Real-time monitoring of body parts and response rate[48], (b) Illustration of human motion sensing system assembled by Bluetooth module and smartphone[62], (c) Composite Materials for Electronic Skin Applications[59], (d) Adhesion test to skin over a long period of time[60]
图 9 (a) 柔性传感器健康监测示意图[58], (b) 实时监测脉搏的反应速度[58], (c) 基于SPRABE的无线监测系统收集心电图和跑步信号的示意图[64], (d) 用于心电图(上)和应变传感(下)模块的皮肤照片[64], (e) 以 2 km·h−1 的速度运行 0、4、8、12、16、20 分钟时的相对阻力变化情况[64]
Figure 9. (a) Flexible Sensor Health Monitoring Schematic[58], (b) Real-time monitoring of pulse response rate[58], (c) Schematic diagram of the SPRABE-skin based wireless monitoring system to collect ECG and running signals[64], (d)Photographs of skin used for ECG (top) and strain sensing (bottom) modules[64], (e) The relative resistance variation at 0, 4, 8, 12, 16, 20 min of 2 km·h−1 running speed[64]
表 1 不同传感原理的优缺点
Table 1. Advantages and disadvantages of different sensing principles
Principle of sensing Advantage Disadvantages Areas of application Piezoresistive Simple structure, easy circuit integration and data processing, flexible structure, and large space for performance adjustment. Less stable, more hysteresis, more affected
by temperatureTactile sensors, pressure sensors Capacitive Simple structure, low temperature influence, low hysteresis, low cost Susceptible to electromagnetic interference, complicated data processing, narrow measurement range Temperature sensors, humidity sensors Piezoelectric Good high-frequency performance, fast response, self-supply of energy Poor static characteristics and small measuring range Energy harvesters, supercapacitors 表 2 WPU柔性传感器制备方法与性能总结
Table 2. Summary of WPU flexible sensor preparation methods and performance
Sensor mechanism Preparation process Conductive filler saturation (physics) response
time9(ms)Special performance bibliography Piezoresistive Screen printing g-MWCNTs GF(~2000) 90 — [37] Capacitive Freeze drying GO、MWCNTs GF(~8.37) — Self-healing [39] Piezoresistive Freeze drying GO GF(~0.2) — — [42] Capacitive Dip coating MXene GF(~960) — — [44] Piezoresistive Casting AgNW — — Self-healing [47] Piezoresistive Casting PANI GF(~168.1) 32 High strength [52] Capacitive Wet spinning PEDOT:PSS — Thermoelectric property [53] Capacitive Casting MXene GF(~474) — — [55] Piezoelectric Vacuum filtration AgNW、MXene GF(~1.6×107) 344 — [56] Piezoresistive Vacuum filtration MXene、ILs GF(~1.8) 185 — [58] Piezoresistive Casting PEDOT:PSS GF(~25) — — [59] Capacitive Dip coating rGO、MWCNT GF(~89) — — [60] Notes: MWCNTs—Multi-walled carbon nanotubes; GO—Graphene oxide; PANI—Polyaniline; PEDOT:PSS—Poly(3,4-ethylenedioxythiophene) /poly(styrenesulfonate); ILs—Ionic liquids: rGO—Reduced graphene oxide. -
[1] RIM Y S, BAE S H, CHEN H, et al. Recent progress in materials and devices toward printable and flexible sensors[J]. Advanced Materials, 2016, 28(22): 4415-4440. doi: 10.1002/adma.201505118 [2] PENG W, WU H. Flexible and stretchable photonic sensors based on modulation of light transmission[J]. Advanced Optical Materials, 2019, 7(12): 1900329. doi: 10.1002/adom.201900329 [3] MENG K, XIAO X, WEI W, et al. Wearable pressure sensors for pulse wave monitoring[J]. Advanced Materials, 2022, 34(21): 2109357. doi: 10.1002/adma.202109357 [4] HAN S T, PENG H, SUN Q, et al. An overview of the development of flexible sensors[J]. Advanced materials, 2017, 29(33): 1700375. doi: 10.1002/adma.201700375 [5] LIAO C, ZHANG M, YAO M Y, et al. Flexible organic electronics in biology: materials and devices[J]. Advanced materials, 2015, 27(46): 7493-7527. doi: 10.1002/adma.201402625 [6] CHOI S, LEE H, GHAFFARI R, et al. Recent advances in flexible and stretchable bio-electronic devices integrated with nanomaterials[J]. Advanced materials, 2016, 28(22): 4203-4218. doi: 10.1002/adma.201504150 [7] BAYER O. Das di-isocyanat-polyadditionsverfahren (polyurethane)[J]. Angewandte Chemie, 1947, 59(9): 257-272. doi: 10.1002/ange.19470590901 [8] 吴景, 曾威, 邝美霞, 等. 细菌纤维素-ZnO/水性聚氨酯复合薄膜的制备与性能[J]. 复合材料学报, 2020, 37(12): 3026-3034.WU Jing, ZENG Wei, KUANG Meixia, et al. Preparation and properties of bacterial cellulose-ZnO/waterborne polyurethane composite films[J]. Acta Materiae Compositae Sinica, 2020, 37(12): 3026-3034(in Chinese). [9] KANG S Y, JI Z, TSENG L F, et al. Design and synthesis of waterborne polyurethanes[J]. Advanced Materials, 2018, 30(18): 1706237. doi: 10.1002/adma.201706237 [10] EPTON R, MCLAREN J. Developments in polymer characterization: Edited by JV Dawkins Applied Science Publishers Ltd, London, 1978, pp 283 [M]. Elsevier. 1979. [11] AKINDOYO J O, BEG M, GHAZALI S, et al. Polyurethane types, synthesis and applications–a review[J]. Rsc Advances, 2016, 6(115): 114453-114482. doi: 10.1039/C6RA14525F [12] DAS A, MAHANWAR P. A brief discussion on advances in polyurethane applications[J]. Advanced Industrial and Engineering Polymer Research, 2020, 3(3): 93-101. doi: 10.1016/j.aiepr.2020.07.002 [13] HU Z, YUAN Q, WANG D. Morphology of waterborne polyurethane/phenolic resin dispersion and the properties of its hybrid film with SiO2[J]. Acta Polymerica Sinica, 2003, (2): 195-200. [14] HONARKAR H. Waterborne polyurethanes: A review[J]. Journal of Dispersion Science and Technology, 2018, 39(4): 507-516. doi: 10.1080/01932691.2017.1327818 [15] LIU X, HONG W, CHEN X. Continuous production of water-borne polyurethanes: A review[J]. Polymers, 2020, 12(12): 2875. doi: 10.3390/polym12122875 [16] 杜峰, 方显力, 项尚林. 内交联型淀粉改性水性聚氨酯固沙剂的制备[J]. 环境科学与技术, 2012, 35(12): 165-168+174.DU Feng, FANG Xianli, XIANG Shanglin, Preparation of Starch Modified Ecological Sand-fixing Agent.[J]. Environmental Science and Technology, 2012, 35(12): 165-168+174(in Chinese) [17] XIA G, HU J, SUN Q, et al. Waterborne polyurethanes with novel chain extenders bearing multiple sulfonate groups[J]. Chemical Engineering Journal, 2023, 478: 147537. doi: 10.1016/j.cej.2023.147537 [18] TANAKA H, KUNIMURA M. Mechanical properties of thermoplastic polyurethanes containing aliphatic polycarbonate soft segments with different chemical structures[J]. Polymer Engineering & Science, 2002, 42(6): 1333-1349. [19] SINGH A, KUMAR R, SONI P K, et al. Investigation of the effect of diisocyanate on the thermal degradation behavior and degradation kinetics of polyether-based polyurethanes[J]. Journal of Macromolecular Science, Part B, 2020, 59(12): 775-795. doi: 10.1080/00222348.2020.1802850 [20] RAHMAN M M. Synthesis and properties of waterborne polyurethane adhesives: effect of chain extender of ethylene diamine, butanediol, and fluoro-butanediol[J]. Journal of adhesion science and technology, 2013, 27(23): 2592-2602. doi: 10.1080/01694243.2013.793996 [21] YEN M S, KUO S C. Effects of mixing procedure on the structure and physical properties of ester–ether-type soft segment waterborne polyurethane[J]. Journal of applied polymer science, 1996, 61(10): 1639-1647. doi: 10.1002/(SICI)1097-4628(19960906)61:10<1639::AID-APP3>3.0.CO;2-H [22] TEE B C, OUYANG J. Soft electronically functional polymeric composite materials for a flexible and stretchable digital future[J]. Advanced Materials, 2018, 30(47): 1802560. doi: 10.1002/adma.201802560 [23] LIU X, TANG C, DU X, et al. A highly sensitive graphene woven fabric strain sensor for wearable wireless musical instruments[J]. Materials Horizons, 2017, 4(3): 477-486. doi: 10.1039/C7MH00104E [24] BOLAND C S, KHAN U, RYAN G, et al. Sensitive electromechanical sensors using viscoelastic graphene-polymer nanocomposites[J]. Science, 2016, 354(6317): 1257-1260. doi: 10.1126/science.aag2879 [25] XU S, FAN Z, LI C, et al. Investigation of strain sensing mechanisms on ultra-thin carbon nanotube networks with different densities[J]. Carbon, 2019, 155: 421-431. doi: 10.1016/j.carbon.2019.09.004 [26] LEE J, KWON H, SEO J, et al. Conductive fiber-based ultrasensitive textile pressure sensor for wearable electronics[J]. Advanced materials, 2015, 27(15): 2433-2439. doi: 10.1002/adma.201500009 [27] TAMBURRANO A, PROIETTI A, FORTUNATO M, et al. Exploring the Capabilities of a Piezoresistive Graphene-Loaded Waterborne Paint for Discrete Strain and Spatial Sensing[J]. Sensors, 2022, 22(11): 4241. doi: 10.3390/s22114241 [28] YING S, LI J, HUANG J, et al. A Flexible Piezocapacitive Pressure Sensor with Microsphere-Array Electrodes[J]. Nanomaterials, 2023, 13(11): 1702. doi: 10.3390/nano13111702 [29] LI Y, SHI L, CHENG Y, et al. Development of conductive materials and conductive networks for flexible force sensors[J]. Chemical Engineering Journal, 2023, 455: 140763. doi: 10.1016/j.cej.2022.140763 [30] YAO Z, FENG H, SHANG K, et al. Skin-like strain sensors based on multiwalled carbon nanotube/polydimethylsiloxane composite films[J]. ACS Applied Nano Materials, 2023, 6(8): 6550-6558. doi: 10.1021/acsanm.3c00083 [31] WANG C, XIA K, WANG H, et al. Advanced carbon for flexible and wearable electronics[J]. Advanced materials, 2019, 31(9): 1801072. doi: 10.1002/adma.201801072 [32] BURMISTROV I, GORSHKOV N, ILINYKH I, et al. Improvement of carbon black based polymer composite electrical conductivity with additions of MWCNT[J]. Composites Science and Technology, 2016, 129: 79-85. doi: 10.1016/j.compscitech.2016.03.032 [33] HE H, ZHONG M, KONKOLEWICZ D, et al. Carbon black functionalized with hyperbranched polymers: synthesis, characterization, and application in reversible CO 2 capture[J]. Journal of Materials Chemistry A, 2013, 1(23): 6810-6821. doi: 10.1039/c3ta10699c [34] CHEN S G, HU J W, ZHANG M Q, et al. Gas sensitivity of carbon black/waterborne polyurethane composites[J]. Carbon, 2004, 42(3): 645-651. doi: 10.1016/j.carbon.2004.01.002 [35] CHEN S G, HU J W, ZHANG M Q, et al. Improvement of gas sensing performance of carbon black/waterborne polyurethane composites: effect of crosslinking treatment[J]. Sensors and Actuators B: Chemical, 2006, 113(1): 361-369. doi: 10.1016/j.snb.2005.03.026 [36] CAO G, HE G, LU L, et al. Hydrogen-bonded n-type waterborne polyurethane/lysine/single-walled carbon nanotube ternary composite: Combining excellent thermoelectric and stretchable properties toward self-powered temperature and strain sensors[J]. Chemical Engineering Journal, 2023, 474: 145664. doi: 10.1016/j.cej.2023.145664 [37] XIA P, LIU P, WU S, et al. Highly stretchable and sensitive flexible resistive strain sensor based on waterborne polyurethane polymer for wearable electronics[J]. Composites Science and Technology, 2022, 221: 109355. doi: 10.1016/j.compscitech.2022.109355 [38] LIU M, LI Z, ZHAO X, et al. Fundamental insights into graphene strain sensing[J]. Nano Letters, 2020, 21(1): 833-839. [39] TU H, ZHOU M, GU Y, et al. Conductive, self-healing, and repeatable graphene/carbon nanotube/polyurethane flexible sensor based on Diels-Alder chemothermal drive[J]. Composites Science and Technology, 2022, 225: 109476. doi: 10.1016/j.compscitech.2022.109476 [40] 马鸿梁, 韩文佳, 景鑫, 等. 纸基柔性导电复合材料的研究进展[J]. 复合材料学报, 2021, 38(8): 2446-2458.MA Hongliang, HAN Wenjia, JING Xin, et al. Research progress of paper-based flexible conductive composite materials[J]. Acta Materiae Compositae Sinica, 2021, 38(8): 2446-2458(in Chinese). [41] KIM M S, RYU K M, LEE S H, et al. Influences of cellulose nanofibril on microstructures and physical properties of waterborne polyurethane-based nanocomposite films[J]. Carbohydrate polymers, 2019, 225: 115233. doi: 10.1016/j.carbpol.2019.115233 [42] ZHAI J, ZHANG Y, CUI C, et al. Flexible waterborne polyurethane/cellulose nanocrystal composite aerogels by integrating graphene and carbon nanotubes for a highly sensitive pressure sensor[J]. ACS Sustainable Chemistry & Engineering, 2021, 9(42): 14029-14039. [43] JASIM S A, HADI J M, OPULENCIA M J C, et al. nanocomposite Electrocatalysts Flame retardancy Energy materials EMI shielding[J]. JOURNAL OF ALLOYS AND COMPOUNDS, 2022, 917(7): 456-472 [44] CAO J, JIANG Y, LI X, et al. A Flexible and Stretchable MXene/Waterborne Polyurethane Composite-Coated Fiber Strain Sensor for Wearable Motion and Healthcare Monitoring[J]. Sensors, 2024, 24(1): 271. doi: 10.3390/s24010271 [45] KANG H, BUCHMAN J T, RODRIGUEZ R S, et al. Stabilization of silver and gold nanoparticles: preservation and improvement of plasmonic functionalities[J]. Chemical reviews, 2018, 119(1): 664-699. [46] WU G, LI J, ZHANG Q. Synergistic effect of multiple hydrogen bond and disulfide bond on healing waterborne conductive polyurethane composite[J]. Polymer, 2022, 258: 125240. doi: 10.1016/j.polymer.2022.125240 [47] NIU H, LI J, SONG X, et al. Multifunctional aqueous polyurethanes with high strength and self-healing efficiency based on silver nanowires for flexible strain sensors[J]. Physical Chemistry Chemical Physics, 2024, 26(3): 2175-2189. doi: 10.1039/D3CP04319C [48] DENG H, CHEN Q, XIE F, et al. Castor oil-based waterborne polyurethane/tunicate cellulose nanocrystals nanocomposites for wearable strain sensors[J]. Carbohydrate Polymers, 2023, 302: 120313. doi: 10.1016/j.carbpol.2022.120313 [49] BAHARFAR M, KALANTAR-ZADEH K. Emerging role of liquid metals in sensing[J]. ACS sensors, 2022, 7(2): 386-408. doi: 10.1021/acssensors.1c02606 [50] ZHANG E, LIU X, LIU Y, et al. Highly stretchable, bionic self-healing waterborne polyurethane elastic film enabled by multiple hydrogen bonds for flexible strain sensors[J]. Journal of Materials Chemistry A, 2021, 9(40): 23055-23071. doi: 10.1039/D1TA05148B [51] ZANARDI C, TERZI F, SEEBER R. Polythiophenes and polythiophene-based composites in amperometric sensing[J]. Analytical and bioanalytical chemistry, 2013, 405: 509-531. doi: 10.1007/s00216-012-6318-7 [52] YU X, YANG H, YE Z, et al. Ultra-Tough Waterborne Polyurethane-Based Graft-Copolymerized Piezoresistive Composite Designed for Rehabilitation Training Monitoring Pressure Sensors[J]. Small, 2023, 2303095. [53] WEN N, FAN Z, YANG S, et al. High-performance stretchable thermoelectric fibers for wearable electronics[J]. Chemical Engineering Journal, 2021, 426: 130816. doi: 10.1016/j.cej.2021.130816 [54] HOFMANN A I, CLOUTET E, HADZIIOANNOU G. Materials for transparent electrodes: from metal oxides to organic alternatives[J]. Advanced Electronic Materials, 2018, 4(10): 1700412. doi: 10.1002/aelm.201700412 [55] WU L, XU C, FAN M, et al. Lotus root structure-inspired Ti3C2-MXene-Based flexible and wearable strain sensor with ultra-high sensitivity and wide sensing range[J]. Composites Part A: Applied Science and Manufacturing, 2022, 152: 106702. doi: 10.1016/j.compositesa.2021.106702 [56] PU J-H, ZHAO X, ZHA X-J, et al. Multilayer structured AgNW/WPU-MXene fiber strain sensors with ultrahigh sensitivity and a wide operating range for wearable monitoring and healthcare[J]. Journal of Materials Chemistry A, 2019, 7(26): 15913-15923. doi: 10.1039/C9TA04352G [57] ZHOU R, LI P, FAN Z, et al. Stretchable heaters with composites of an intrinsically conductive polymer, reduced graphene oxide and an elastomer for wearable thermotherapy[J]. Journal of Materials Chemistry C, 2017, 5(6): 1544-1551. doi: 10.1039/C6TC04849H [58] WANG S, CHENG H, YAO B, et al. Self-adhesive, stretchable, biocompatible, and conductive nonvolatile eutectogels as wearable conformal strain and pressure sensors and biopotential electrodes for precise health monitoring[J]. ACS Applied Materials & Interfaces, 2021, 13(17): 20735-20745. [59] XU S, FAN Z, YANG S, et al. Flexible, self-powered and multi-functional strain sensors comprising a hybrid of carbon nanocoils and conducting polymers[J]. Chemical Engineering Journal, 2021, 404: 126064. doi: 10.1016/j.cej.2020.126064 [60] WANG S, FANG Y, HE H, et al. Wearable stretchable dry and self-adhesive strain sensors with conformal contact to skin for high-quality motion monitoring[J]. Advanced Functional Materials, 2021, 31(5): 2007495. doi: 10.1002/adfm.202007495 [61] LIN M, ZHENG Z, YANG L, et al. A high-performance, sensitive, wearable multifunctional sensor based on rubber/CNT for human motion and skin temperature detection[J]. Advanced Materials, 2022, 34(1): 2107309. doi: 10.1002/adma.202107309 [62] WEN X, DENG Z, WANG H, et al. High strength, self-healing sensitive ionogel sensor based on MXene/ionic liquid synergistic conductive network for human-motion detection[J]. Journal of Materials Chemistry B, 2023, 11(47): 11251-11264. doi: 10.1039/D3TB01570J [63] HEIDARI H. Electronic skins with a global attraction[J]. Nature Electronics, 2018, 1(11): 578-579. doi: 10.1038/s41928-018-0165-2 [64] HAO Y, YAN Q, LIU H, et al. A Stretchable, Breathable, And Self-Adhesive Electronic Skin with Multimodal Sensing Capabilities for Human-Centered Healthcare[J]. Advanced Functional Materials, 2023, 33(44): 2303881. doi: 10.1002/adfm.202303881 -
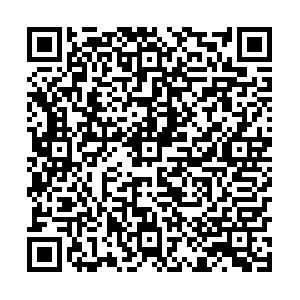
计量
- 文章访问数: 178
- HTML全文浏览量: 110
- 被引次数: 0