Progress of biomass materials in triboelectric flexible sensors
-
摘要: 生物质摩擦电柔性传感器是以易降解、生物相容性好的生物质材料为基材,由正负摩擦层组成,不需要外接电源的柔性传感器,其具有便捷灵活、灵敏、可再生等特点,在人体运动监测、医疗健康、软体机器人等领域得到了全面的发展。本文首先介绍了基于不同供电原理的自供电柔性传感器的分类,包括压电型自供电柔性传感器、热电型自供电柔性传感器、摩擦电型自供电柔性传感器以及光电型自供电柔性传感器,其中重点介绍摩擦电型;进一步综述了各类生物质材料在摩擦电柔性传感器中的研究,包括纤维素、壳聚糖、木质素、海藻酸钠、胶原纤维等;然后讨论了摩擦电柔性传感器的结构类型,如水凝胶型、气凝胶型、薄膜型。最后对生物质摩擦电柔性传感器的材料选择、设计类型、附加性能和应用前景等进行了展望。Abstract: Biomass friction electric flexible sensors are flexible sensors based on easily degradable and biocompatible biomass materials, consisting of positive and negative friction layers, and do not require external power supply, which are convenient, flexible, sensitive, renewable, etc., and have been comprehensively developed in the fields of human body movement monitoring, e-skinning and soft robotics. This paper firstly introduces the classification of self-powered flexible sensors based on different power supply principles, including piezoelectric self-powered flexible sensors, thermoelectric self-powered flexible sensors, friction electric self-powered flexible sensors and photoelectric self-powered flexible sensors, with a focus on the friction electric type. A further overview is given on the study of various types of biomass materials in friction electric flexible sensors, including cellulose, chitosan, lignin, sodium alginate and collagen fibres. Then the structure types of friction electric flexible sensors, such as hydrogel type, aerogel type, and film type, are discussed. Finally, the material selection, design types, additional properties and application prospects of biomass friction electric flexible sensors are prospected.
-
Key words:
- self-powered /
- triboelectricity /
- biomass materials /
- flexible sensors /
- structure of sensors
-
表 1 水凝胶、气凝胶、薄膜柔性传感器的优劣势对比图
Table 1. Comparison of advantages and disadvantages of hydrogel, aerogel and thin film flexible sensors
类型 优势 劣势 水凝胶 机械性能好(可拉伸、韧性) 易失水、持久性不佳、稳
定性差气凝胶 孔隙率高、密度低、质轻、导热率低 机械性能受限、制备工艺复杂 薄膜 灵活、制备简单 透气性、耐磨性不佳 -
[1] HUANG J, LI D, ZHAO M, et al. Flexible electrically conductive biomass-based aerogels for piezoresistive pressure/strain sensors[J]. Chemical Engineering Journal, 2019, 373: 1357-1366. doi: 10.1016/j.cej.2019.05.136 [2] LI J, LI W, LIN J, et al. Multi-modal piezoresistive sensor based on cotton fiber aerogel/PPy for sound detection and respiratory monitoring[J]. Composites Science and Technology, 2023, 235: 109953. doi: 10.1016/j.compscitech.2023.109953 [3] WANG G, LIU X, SONG Z, et al. Multifunctional flexible pressure sensor based on a cellulose fiber-derived hierarchical carbon aerogel[J]. ACS Applied Electronic Materials, 2023, 5(3): 1581-1591. doi: 10.1021/acsaelm.2c01628 [4] WANG X, YUE O, LIU X, et al. A novel bio-inspired multi-functional collagen aggregate based flexible sensor with multi-layer and internal 3D network structure[J]. Chemical Engineering Journal, 2020, 392: 123672. doi: 10.1016/j.cej.2019.123672 [5] YANG J, SUN X, KANG Q, et al. Freezing-tolerant and robust gelatin-based supramolecular conductive hydrogels with double-network structure for wearable sensors[J]. Polymer Testing, 2021, 93: 106879. doi: 10.1016/j.polymertesting.2020.106879 [6] LONG S, FENG Y, HE F, et al. Biomass-derived, multifunctional and wave-layered carbon aerogels toward wearable pressure sensors, supercapacitors and triboelectric nanogenerators[J]. Nano Energy, 2021, 85: 105973. doi: 10.1016/j.nanoen.2021.105973 [7] ZHANG G, LIAO Q, MA M, et al. Uniformly assembled vanadium doped ZnO microflowers/bacterial cellulose hybrid paper for flexible piezoelectric nanogenerators and self-powered sensors[J]. Nano Energy, 2018, 52: 501-509. doi: 10.1016/j.nanoen.2018.08.020 [8] LV P, ZHOU H, MENSAH A, et al. A highly flexible self-powered biosensor for glucose detection by epitaxial deposition of gold nanoparticles on conductive bacterial cellulose[J]. Chemical Engineering Journal, 2018, 351: 177-188. doi: 10.1016/j.cej.2018.06.098 [9] LI Y, CHEN S, YAN H, et al. Biodegradable, transparent, and antibacterial alginate-based triboelectric nanogenerator for energy harvesting and tactile sensing[J]. Chemical Engineering Journal, 2023, 468: 143572. doi: 10.1016/j.cej.2023.143572 [10] 李鸿冰, 韩文佳, 姜亦飞, 等. 柔性自供电传感器的研究进展[J]. 电子元件与材料, 2020, 39(8): 1-12.LI Hong-Bing, HAN Wen-Jia, JIANG Yi-Fei, et al. Research progress of flexible self-powered sensor[J]. Electronic Components and Materials, 2020, 39(8): 1-12(in Chinese). [11] LIN G L, LIN A X, LIU M Y, et al. Barium titanate–bismuth ferrite/polyvinylidene fluoride nanocomposites as flexible piezoelectric sensors with excellent thermal stability[J]. Sensors and Actuators A: Physical, 2022, 346: 113885. doi: 10.1016/j.sna.2022.113885 [12] LIU X, TONG J, WANG J, et al. BaTiO 3/MXene/PVDF-TrFE composite films via an electrospinning method for flexible piezoelectric pressure sensors[J]. Journal of Materials Chemistry C, 2023, 11(14): 4614-4622. doi: 10.1039/D2TC05291A [13] QI F, XU L, HE Y, et al. PVDF-Based Flexible Piezoelectric Tactile Sensors[J]. Crystal Research and Technology, 2023, 58(10): 2300119. doi: 10.1002/crat.202300119 [14] WAN X, CONG H, JIANG G, et al. A review on PVDF nanofibers in textiles for flexible piezoelectric sensors[J]. ACS Applied Nano Materials, 2023, 6(3): 1522-1540. doi: 10.1021/acsanm.2c04916 [15] SASIKUMAR R, CHO S, WAQAR A, et al. Microcrack-assisted piezoelectric acoustic sensor based on f-MWCNTs/BaTiO3@ PDMS nanocomposite and its self-powered voice recognition applications[J]. Chemical Engineering Journal, 2024, 479: 147297. doi: 10.1016/j.cej.2023.147297 [16] GAO X, ZHENG M, YAN X, et al. The alignment of BCZT particles in PDMS boosts the sensitivity and cycling reliability of a flexible piezoelectric touch sensor[J]. Journal of Materials Chemistry C, 2019, 7(4): 961-967. doi: 10.1039/C8TC04741C [17] ZHANG Y, GE B, FENG J, et al. High-performance self-powered flexible Tthermoelectric device for accelerated wound healing[J]. Advanced Functional Materials. 2024: 2403990. [18] LI Y, ZHANG Q, LIU K, et al. Multi-scale hierarchical microstructure modulation towards high room temperature thermoelectric performance in n-type Bi2Te3-based alloys[J]. Materials Today Nano, 2023, 22: 100340. doi: 10.1016/j.mtnano.2023.100340 [19] LI X, LU Y, CAI K, et al. Exceptional power factor of flexible Ag/Ag2Se thermoelectric composite films[J]. Chemical Engineering Journal, 2022, 434: 134739. doi: 10.1016/j.cej.2022.134739 [20] ZHENG C, XIANG L, JIN W, et al. A flexible self-powered sensing element with integrated organic thermoelectric generator[J]. Advanced Materials Technologies, 2019, 4(8): 1900247. doi: 10.1002/admt.201900247 [21] ZHU P, WANG Y, SHENG M, et al. A flexible active dual-parameter sensor for sensitive temperature and physiological signal monitoring via integrating thermoelectric and piezoelectric conversion[J]. Journal of materials chemistry A, 2019, 7(14): 8258-8267. doi: 10.1039/C9TA00682F [22] DU M, OUYANG J, ZHANG K. Flexible Bi2Te 3/PEDOT nanowire sandwich-like film towards high-performance wearable cross-plane thermoelectric generator and temperature sensor array[J]. Journal of Materials Chemistry A, 2023, 11(30): 16039-16048 doi: 10.1039/D3TA02876C [23] KONSTANTATOS G. Current status and technological prospect of photodetectors based on two-dimensional materials[J]. Nature communications, 2018, 9(1): 5266. doi: 10.1038/s41467-018-07643-7 [24] MALLEM S P R, PUNEETHA P, LEE D, et al. Light-sensitive and strain-controlled flexible DNA/graphene/GaN bio-hybrid sensor based on the piezophototronic effect[J]. Nano Energy, 2023, 116: 108807. doi: 10.1016/j.nanoen.2023.108807 [25] SUN J, HUA Q, ZHOU R, et al. Piezo-phototronic effect enhanced efficient flexible perovskite solar cells[J]. ACS Nano, 2019, 13(4): 4507-4513. doi: 10.1021/acsnano.9b00125 [26] KIM Y K, HWANG S, KIM S, et al. ZnO nanostructure electrodeposited on flexible conductive fabric: A flexible photo-sensor[J]. Sensors and Actuators B: Chemical, 2017, 240: 1106-1113. doi: 10.1016/j.snb.2016.09.072 [27] SUN H, TIAN W, CAO F, et al. Ultrahigh-performance self-powered flexible double-twisted fibrous broadband perovskite photodetector[J]. Advanced Materials, 2018, 30(21): 1706986. doi: 10.1002/adma.201706986 [28] ZHANG H, ZHANG X, LIU C, et al. High-responsivity, high-detectivity, ultrafast topological insulator Bi2Se3/silicon heterostructure broadband photodetectors[J]. ACS Nano, 2016, 10(5): 5113-5122. doi: 10.1021/acsnano.6b00272 [29] XIAO J, ZHANG Z, WANG S, et al. High-Performance thermoelectric generator based on n-Type flexible composite and its application in Self-Powered temperature sensor[J]. Chemical Engineering Journal, 2024, 479: 147569. doi: 10.1016/j.cej.2023.147569 [30] WANG Z L. Triboelectric nanogenerator (TENG)—sparking an energy and sensor revolution[J]. Advanced Energy Materials, 2020, 10(17): 2000137. doi: 10.1002/aenm.202000137 [31] LIN M, CHANG P, LEE C, et al. Biowaste eggshell membranes for bio-triboelectric nanogenerators and smart sensors[J]. ACS omega, 2023, 8(7): 6699-6707. doi: 10.1021/acsomega.2c07292 [32] APPAMATO I, BUNRIW W, HARNCHANA V, et al. Engineering triboelectric charge in natural rubber–Ag nanocomposite for enhancing electrical output of a triboelectric nanogenerator[J]. ACS Applied Materials & Interfaces, 2022, 15(1): 973-983. [33] BEHERA S A, PANDA S, HAJRA S, et al. EVA/PZT-composite-based triboelectric nanogenerator for energy harvesting[J]. Energy Technology, 2023, 11(9): 2300469. doi: 10.1002/ente.202300469 [34] SHIN J, JI S, CHO H, et al. Highly flexible triboelectric nanogenerator using porous carbon nanotube composites[J]. Polymers, 2023, 15(5): 1135. doi: 10.3390/polym15051135 [35] SHI X, WEI Y, YAN R, et al. Leaf surface-microstructure inspired fabrication of fish gelatin-based triboelectric nanogenerator[J]. Nano Energy, 2023, 109: 108231. doi: 10.1016/j.nanoen.2023.108231 [36] ZHANG R, DAHLSTROM C, ZOU H, et al. Cellulose-based fully green triboelectric nanogenerators with output power density of 300 W m−2[J]. Advanced Materials, 2020, 32(38): 2002824. doi: 10.1002/adma.202002824 [37] CHEN S, SONG Y, DING D, et al. Flexible and anisotropic strain sensor based on carbonized crepe paper with aligned cellulose fibers[J]. Advanced Functional Materials, 2018, 28(42): 1802547. doi: 10.1002/adfm.201802547 [38] ZHANG J, HU S, SHI Z, et al. Eco-friendly and recyclable all cellulose triboelectric nanogenerator and self-powered interactive interface[J]. Nano Energy, 2021, 89: 106354. doi: 10.1016/j.nanoen.2021.106354 [39] WANG Z, CHEN C, FANG L, et al. Biodegradable, conductive, moisture-proof, and dielectric enhanced cellulose-based triboelectric nanogenerator for self-powered human-machine interface sensing[J]. Nano Energy, 2023, 107: 108151. doi: 10.1016/j.nanoen.2022.108151 [40] XU M, WU T, SONG Y, et al. Achieving high-performance energy harvesting and self-powered sensing in a flexible cellulose nanofibril/MoS2/BaTiO3 composite piezoelectric nanogenerator[J]. Journal of Materials Chemistry C, 2021, 9(43): 15552-15565. doi: 10.1039/D1TC03886A [41] YAO C, YIN X, YU Y, et al. Chemically functionalized natural cellulose materials for effective triboelectric nanogenerator development[J]. Advanced Functional Materials, 2017, 27(30): 1700794. doi: 10.1002/adfm.201700794 [42] MI H, JING X, ZHENG Q, et al. High-performance flexible triboelectric nanogenerator based on porous aerogels and electrospun nanofibers for energy harvesting and sensitive self-powered sensing[J]. Nano Energy, 2018, 48: 327-336. doi: 10.1016/j.nanoen.2018.03.050 [43] KUMAR M N R. A review of chitin and chitosan applications[J]. Reactive and functional polymers, 2000, 46(1): 1-27. doi: 10.1016/S1381-5148(00)00038-9 [44] YAO C, HERNANDEZ A, YU Y, et al. Triboelectric nanogenerators and power-boards from cellulose nanofibrils and recycled materials[J]. Nano Energy, 2016, 30: 103-108. doi: 10.1016/j.nanoen.2016.09.036 [45] LU Y, XIANG H, JIE Y, et al. Antibacterial Triboelectric Nanogenerator for Mite Removal and Intelligent Human Monitoring[J]. Advanced Materials Technologies, 2023, 8(16): 2300192. doi: 10.1002/admt.202300192 [46] ZHENG Z, YU D, WANG B, et al. Ultrahigh sensitive, eco-friendly, transparent triboelectric nanogenerator for monitoring human motion and vehicle movement[J]. Chemical Engineering Journal, 2022, 446: 137393. doi: 10.1016/j.cej.2022.137393 [47] ZHANG Y, SHAO Y, LUO C, et al. Preparation of a high-performance chitosan-based triboelectric nanogenerator by regulating the surface microstructure and dielectric constant[J]. Journal of Materials Chemistry C, 2023, 11(1): 260-268. doi: 10.1039/D2TC04262B [48] GAO C, TONG W, LIU S, et al. Fully degradable chitosan–based triboelectric nanogenerators applying in disposable medical products for information transfer[J]. Nano Energy, 2023, 117: 108876. doi: 10.1016/j.nanoen.2023.108876 [49] CHAROONSUK T, PONGAMPAI S, PALAWANIT P, et al. Achieving a highly efficient chitosan-based triboelectric nanogenerator via adding organic proteins: Influence of morphology and molecular structure[J]. Nano Energy, 2021, 89: 106430. doi: 10.1016/j.nanoen.2021.106430 [50] ZHANG X, LIU W, CAI J, et al. Equip the hydrogel with armor: strong and super tough biomass reinforced hydrogels with excellent conductivity and anti-bacterial performance[J]. Journal of Materials Chemistry A, 2019, 7(47): 26917-26926. doi: 10.1039/C9TA10509C [51] JIANG L, LIU J, HE S, et al. Flexible wearable sensors based on lignin doped organohydrogels with multi-functionalities[J]. Chemical Engineering Journal, 2022, 430: 132653. doi: 10.1016/j.cej.2021.132653 [52] TANGUY N R, KAZEMI K K, HONG J, et al. Flexible, robust, and high-performance gas sensors based on lignocellulosic nanofibrils[J]. Carbohydrate Polymers, 2022, 278: 118920. doi: 10.1016/j.carbpol.2021.118920 [53] FUNAYAMA R, HAYASHI S, TERAKAWA M. Laser-induced graphitization of lignin/PLLA composite sheets for biodegradable triboelectric nanogenerators[J]. ACS Sustainable Chemistry & Engineering, 2023, 11(7): 3114-3122. [54] JO H, PARK D, JOO M, et al. Performance-enhanced eco-friendly triboelectric nanogenerator via wettability manipulation of lignin[J]. EcoMat, 2023, 5(11): 12413. doi: 10.1002/eom2.12413 [55] CHEN W, LI C, TAO Y, et al. Modulating spatial charge distribution of lignin for eco-friendly and recyclable triboelectric nanogenerator[J]. Nano Energy, 2023, 116: 108802. doi: 10.1016/j.nanoen.2023.108802 [56] PANG Y, XI F, LUO J, et al. An alginate film-based degradable triboelectric nanogenerator[J]. RSC advances, 2018, 8(12): 6719-6726. doi: 10.1039/C7RA13294H [57] SAQIB Q M, CHOUGALE M Y, KHAN M U, et al. Natural seagrass tribopositive material based spray coatable triboelectric nanogenerator[J]. Nano Energy, 2021, 89: 106458. doi: 10.1016/j.nanoen.2021.106458 [58] SAQIB Q M, CHOUGALE M Y, KHAN M U, et al. Natural seagrass tribopositive material based spray coatable triboelectric nanogenerator[J]. Nano Energy, 2021, 89: 106458. doi: 10.1016/j.nanoen.2021.106458 [59] LI Y, CHEN S, YAN H, et al. Biodegradable, transparent, and antibacterial alginate-based triboelectric nanogenerator for energy harvesting and tactile sensing[J]. Chemical Engineering Journal, 2023, 468: 143572. doi: 10.1016/j.cej.2023.143572 [60] ZHAO L, LING Q, FAN X, et al. Self-healable, adhesive, anti-drying, freezing-tolerant, and transparent conductive organohydrogel as flexible strain sensor, triboelectric nanogenerator, and skin barrier[J]. ACS Applied Materials & Interfaces, 2023, 15(34): 40975-40990. [61] SHENG F, YI J, SHEN S, et al. Self-powered smart arm training band sensor based on extremely stretchable hydrogel conductors[J]. ACS Applied Materials & Interfaces, 2021, 13(37): 44868-44877. [62] MA J, PAN Z, ZHANG W, et al. High-sensitivity microchannel-structured collagen fiber-based sensors with antibacterial and hydrophobic properties[J]. ACS Sustainable Chemistry & Engineering, 2022, 10(50): 16814-16824. [63] ZHANG X, YANG Y, YAO J, et al. Strong collagen hydrogels by oxidized dextran modification[J]. ACS Sustainable Chemistry & Engineering, 2014, 2(5): 1318-1324. [64] SONG B, FAN X, SHEN J, et al. Ultra-stable and self-healing coordinated collagen-based multifunctional double-network organohydrogel e-skin for multimodal sensing monitoring of strain-resistance, bioelectrode, and self-powered triboelectric nanogenerator[J]. Chemical Engineering Journal, 2023, 474: 145780. doi: 10.1016/j.cej.2023.145780 [65] ZHANG S, XIAO Y, CHEN H, et al. Flexible triboelectric tactile sensor based on a robust MXene/leather film for human–machine interaction[J]. ACS Applied Materials & Interfaces, 2023, 15(10): 13802-13812. [66] WANG L, XU T, ZHANG X. Multifunctional conductive hydrogel-based flexible wearable sensors[J]. TrAC Trends in Analytical Chemistry, 2021, 134: 116130. doi: 10.1016/j.trac.2020.116130 [67] OH B, LIM Y, KO K W, et al. Ultra-soft and highly stretchable tissue-adhesive hydrogel based multifunctional implantable sensor for monitoring of overactive bladder[J]. Biosensors and Bioelectronics, 2023, 225: 115060. doi: 10.1016/j.bios.2023.115060 [68] HAN Y, WEI H, DU Y, et al. Ultrasensitive flexible thermal sensor arrays based on high-thermopower ionic thermoelectric hydrogel[J]. Advanced Science, 2023, 10(25): 2302685. doi: 10.1002/advs.202302685 [69] LIANG Y, YE L, SUN X, et al. Tough and stretchable dual ionically cross-linked hydrogel with high conductivity and fast recovery property for high-performance flexible sensors[J]. ACS applied materials & interfaces, 2019, 12(1): 1577-1587. [70] TIE J, CHAI H, MAO Z, et al. Nanocellulose-mediated transparent high strength conductive hydrogel based on in-situ formed polypyrrole nanofibrils as a multimodal sensor[J]. Carbohydrate Polymers, 2021, 273: 118600. doi: 10.1016/j.carbpol.2021.118600 [71] PAN L, HAN L, LIU H, et al. Flexible sensor based on Hair-like microstructured ionic hydrogel with high sensitivity for pulse wave detection[J]. Chemical Engineering Journal, 2022, 450: 137929. doi: 10.1016/j.cej.2022.137929 [72] GUO W, MAI T, HUANG L, et al. Multifunctional MXene conductive zwitterionic hydrogel for flexible wearable sensors and arrays[J]. ACS Applied Materials & Interfaces, 2023, 15(20): 24933-24947. [73] LI T, WEI H, ZHANG Y, et al. Sodium alginate reinforced polyacrylamide/xanthan gum double network ionic hydrogels for stress sensing and self-powered wearable device applications[J]. Carbohydrate Polymers, 2023, 309: 120678. doi: 10.1016/j.carbpol.2023.120678 [74] FENG Y, YU J, SUN D, et al. Extreme environment-adaptable and fast self-healable eutectogel triboelectric nanogenerator for energy harvesting and self-powered sensing[J]. Nano Energy, 2022, 98: 107284. doi: 10.1016/j.nanoen.2022.107284 [75] CHU Y, FAN Q, CHAI C, et al. “Water-in-deep eutectic solvent” gel electrolytes synergistically controlled by solvation regulation and gelation strategies for flexible electronic devices[J]. ACS Applied Materials & Interfaces, 2023, 15(9): 12088-12098. [76] YANG J, TIAN X, FAN J, et al. Extreme condition-resistant flexible triboelectric nanogenerator and sensor based on swollen-yet-enhanced and self-healing deep eutectic gel[J]. Polymer, 2023, 283: 126238. doi: 10.1016/j.polymer.2023.126238 [77] WANG Z, CONG Y, FU J. Stretchable and tough conductive hydrogels for flexible pressure and strain sensors[J]. Journal of materials chemistry B, 2020, 8(16): 3437-3459. doi: 10.1039/C9TB02570G [78] WANG Z, CONG Y, FU J. Stretchable and tough conductive hydrogels for flexible pressure and strain sensors[J]. Journal of materials chemistry B, 2020, 8(16): 3437-3459. doi: 10.1039/C9TB02570G [79] SHENG F, YI J, SHEN S, et al. Self-powered smart arm training band sensor based on extremely stretchable hydrogel conductors[J]. ACS Applied Materials & Interfaces, 2021, 13(37): 44868-44877. [80] MOGLI G, REINA M, CHIAPPONE A, et al. Self-powered integrated tactile sensing system based on ultrastretchable, self-healing and 3D printable ionic conductive hydrogel[J]. Advanced Functional Materials, 2023, 34: 2307133. [81] YANG B, YUAN W. Highly stretchable and transparent double-network hydrogel ionic conductors as flexible thermal–mechanical dual sensors and electroluminescent devices[J]. ACS applied materials & interfaces, 2019, 11(18): 16765-16775. [82] ZHANG H, XUE K, XU X, et al. Green and low-cost alkali-polyphenol synergetic self-catalysis system access to fast gelation of self-healable and self-adhesive conductive hydrogels for self-powered triboelectric nanogenerators[J]. Small, 2023, 20(10): 2305502. [83] MA C, XIE F, WEI L, et al. All-starch-based hydrogel for flexible electronics: strain-sensitive batteries and self-powered sensors[J]. ACS Sustainable Chemistry & Engineering, 2022, 10(20): 6724-6735. [84] SUN H, XU Z, GAO C. Multifunctional, ultra-flyweight, synergistically assembled carbon aerogels[J]. Advanced materials, 2013, 25(18): 2554-2560. doi: 10.1002/adma.201204576 [85] NARDECCHIA S, CARRIAZO D, FERRER M L, et al. Three dimensional macroporous architectures and aerogels built of carbon nanotubes and/or graphene: synthesis and applications[J]. Chemical Society Reviews, 2013, 42(2): 794-830. doi: 10.1039/C2CS35353A [86] ZHU W, CHEN M, JANG J, et al. Amino-functionalized nanocellulose aerogels for the superior adsorption of CO2 and separation of CO2/CH4 mixture gas[J]. Carbohydrate Polymers, 2024, 323: 121393. doi: 10.1016/j.carbpol.2023.121393 [87] LONG S, FENG Y, HE F, et al. An ultralight, supercompressible, superhydrophobic and multifunctional carbon aerogel with a specially designed structure[J]. Carbon, 2020, 158: 137-145. doi: 10.1016/j.carbon.2019.11.065 [88] ZHANG C, LIU S, QI Y, et al. Conformal carbon coated TiO2 aerogel as superior anode for lithium-ion batteries[J]. Chemical Engineering Journal, 2018, 351: 825-831. doi: 10.1016/j.cej.2018.06.125 [89] YAVARI F, CHEN Z, THOMAS A V, et al. High sensitivity gas detection using a macroscopic three-dimensional graphene foam network[J]. Scientific reports, 2011, 1(1): 166. doi: 10.1038/srep00166 [90] LONG S, FENG Y, HE F, et al. Biomass-derived, multifunctional and wave-layered carbon aerogels toward wearable pressure sensors, supercapacitors and triboelectric nanogenerators[J]. Nano Energy, 2021, 85: 105973. doi: 10.1016/j.nanoen.2021.105973 [91] CHENG Y, ZHU W, LU X, et al. Lightweight and flexible MXene/carboxymethyl cellulose aerogel for electromagnetic shielding, energy harvest and self-powered sensing[J]. Nano Energy, 2022, 98: 107229. doi: 10.1016/j.nanoen.2022.107229 [92] CHO Y, LEE K, PARK S, et al. Rotational wind power triboelectric nanogenerator using aerodynamic changes of friction area and the adsorption effect of hematoxylin onto feather based on a diversely evolved hyper-branched structure[J]. Nano Energy, 2019, 61: 370-380. doi: 10.1016/j.nanoen.2019.04.083 [93] PENG X, DONG K, ZHANG Y, et al. Sweat-permeable, biodegradable, transparent and self-powered chitosan-based electronic skin with ultrathin elastic gold nanofibers[J]. Advanced Functional Materials, 2022, 32(20): 2112241. doi: 10.1002/adfm.202112241 [94] ZHANG M, TAN Z, ZHANG Q, et al. Flexible self-powered friction piezoelectric sensor based on structured PVDF-based composite nanofiber membranes[J]. ACS Applied Materials & Interfaces, 2023, 32(20): 2112241. [95] ZHANG J, HU Y, LIN X, et al. High-performance triboelectric nanogenerator based on chitin for mechanical-energy harvesting and self-powered sensing[J]. Carbohydrate Polymers, 2022, 291: 119586. doi: 10.1016/j.carbpol.2022.119586 [96] LI Y, CHEN S, YAN H, et al. Biodegradable, transparent, and antibacterial alginate-based triboelectric nanogenerator for energy harvesting and tactile sensing[J]. Chemical Engineering Journal, 2023, 468: 143572. doi: 10.1016/j.cej.2023.143572 [97] YAN J, LV M, QIN Y, et al. Triboelectric nanogenerators based on membranes comprised of polyurethane fibers loaded with ethyl cellulose and barium titanate nanoparticles[J]. ACS Applied Nano Materials, 2023, 6(7): 5675-5684. doi: 10.1021/acsanm.3c00124 [98] JIANG C, LI X, YAO Y, et al. A multifunctional and highly flexible triboelectric nanogenerator based on MXene-enabled porous film integrated with laser-induced graphene electrode[J]. Nano Energy, 2019, 66: 104121. doi: 10.1016/j.nanoen.2019.104121 [99] ZHANG Y, SHAO Y, LUO C, et al. Preparation of a high-performance chitosan-based triboelectric nanogenerator by regulating the surface microstructure and dielectric constant[J]. Journal of Materials Chemistry C, 2023, 11(1): 260-268. doi: 10.1039/D2TC04262B [100] CHEN K, LI Y, YANG G, et al. Fabric-based TENG woven with bio-fabricated superhydrophobic bacterial cellulose fiber for energy harvesting and motion detection[J]. Advanced Functional Materials, 2023, 33(45): 2304809. doi: 10.1002/adfm.202304809 [101] YI F, ZHANG Z, KANG Z, et al. Recent advances in triboelectric nanogenerator-based health monitoring[J]. Advanced Functional Materials, 2019, 29(41): 1808849. doi: 10.1002/adfm.201808849 [102] YUE J, TENG Y, HUANG Y, et al. Reduced graphene oxide interlocked carbonized loofah for energy harvesting and physiological signal monitoring[J]. ACS Applied Electronic Materials. 2024. [103] GAO Y, XU B, TAN D, et al. Asymmetric-elastic-structure fabric-based triboelectric nanogenerators for wearable energy harvesting and human motion sensing[J]. Chemical Engineering Journal, 2023, 466: 143079. doi: 10.1016/j.cej.2023.143079 [104] LIU W, YU W, LI K, et al. Enhancing blind-dumb assistance through a self-powered tactile sensor-based Braille typing system[J]. Nano Energy, 2023, 116: 108795. doi: 10.1016/j.nanoen.2023.108795 [105] YUAN Y, LIU B, LI H, et al. Flexible wearable sensors in medical monitoring[J]. Biosensors, 2022, 12(12): 1069. doi: 10.3390/bios12121069 [106] LI C, XU Z, XU S, et al. Miniaturized retractable thin-film sensor for wearable multifunctional respiratory monitoring[J]. Nano Research, 2023, 16(6): 11846-11854. [107] SHI X, CHEN P, HAN K, et al. A strong, biodegradable, and recyclable all-lignocellulose fabricated triboelectric nanogenerator for self-powered disposable medical monitoring[J]. Journal of Materials Chemistry A, 2023, 11(22): 11730. doi: 10.1039/D3TA01763J [108] JIANG W, LI H, LIU Z, et al. Fully bioabsorbable natural-materials-based triboelectric nanogenerators[J]. Advanced Materials, 2018, 30(32): 1801895. doi: 10.1002/adma.201801895 [109] JIAN G, ZHU S, YUAN X, et al. Biodegradable triboelectric nanogenerator as a implantable power source for embedded medicine devices[J]. NPG Asia Materials, 2024, 16(1): 12. doi: 10.1038/s41427-023-00528-2 [110] ZHANG Z, CAI J. A triboelectric joint sensor imitating soft robot for human joint rehabilitation monitoring[J]. Nano, 2022, 17(8): 2250059. doi: 10.1142/S179329202250059X [111] ZHANG S, MENG S, ZHANG K, et al. A high-performance s-TENG based on the synergistic effect of keratin and calcium chloride for finger activity tracking[J]. Nano Energy, 2023, 112: 108443. doi: 10.1016/j.nanoen.2023.108443 -
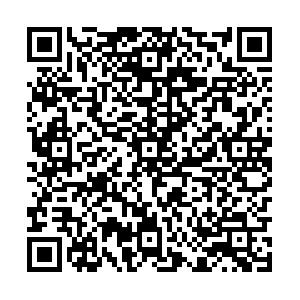
计量
- 文章访问数: 82
- HTML全文浏览量: 32
- 被引次数: 0