Effects of Y element and dual-step rolling on microstructure and mechanical properties of CuAlMn alloy
-
摘要: Cu基形状记忆合金由于晶粒粗大和弹性各向异性,呈现出较低的力学断裂强度和塑性形变能力。本文通过添加微量稀土Y元素制备了系列Cu-11.36Al-5Mn合金,并经热轧和双步轧制(热轧+冷轧)实现了对合金微观结构的调控。实验发现,CuAlMn合金由奥氏体和少量的18R马氏体组成。Y元素添加后,晶粒得到显著细化,基体中可见沿晶分布的含Y沉淀析出相以及伴生的富Al相,热轧形变后晶粒进一步细化,高密度位错和位错胞元结构出现。双步轧制退火后,位错密度持续增大并出现了高密度位错缠结,沿晶析出大量富铜 沉淀相,孪晶和马氏体条交替排列。拉伸力学性能测试表明,稀土Y元素可显著提升合金的力学性能,热轧和双步轧制后进一步提高,拉伸断裂强度从366.67 MPa (原始态)→546.99 MPa (0.4% Y )→879.25 MPa (80%热轧)→
1025.25 MPa (60%热轧-60%冷轧)。断后伸长率与拉伸断裂强度具有相同的变化趋势,从原始态的3.05%提升至双步轧制形变后的8.38%。最大超弹性应变随Y元素的添加而增大,但相同应变下原始态CuAlMn具有更高的超弹性,且经历轧制形变后超弹性应变迅速下降。最后,通过微观结构的演变系统讨论了合金力学性能提升机制。Abstract: Cu-based shape memory alloys exhibit lower mechanical fracture strength and plastic deformation capability due to the coarse grain size and elastic anisotropy. A series of Cu-11.36Al-5Mn alloys were prepared by adding trace amounts of the rare earth element Y, and the microstructure control was achieved through hot rolling and dual-step rolling (hot rolling + cold rolling). It is revealed that the CuAlMn alloy is composed of austenite and a small amount of 18R martensite. A significant refinement of grains was obtained after the addition of Y element. Besides, Y-containing precipitates and Al-rich phases were observed to distribute along the grain boundary within the matrix. After hot rolling deformation, the alloy grains further refined and high-density dislocations and dislocation cell structures appeared. The dislocation density continued to increase accompanied by the emergence of high-density dislocation tangles after dual-step rolling and subsequent annealing. Abundant Cu-rich precipitates precipitated along the grain boundaries, with alternating arrangements of twins and martensite laths. Tensile mechanical property tests show that the mechanical properties are significantly enhanced due to the inclusion of the rare earth element Y, with further improvement after rolling deformation. The tensile fracture strength increases from 366.67 MPa (as-received condition) → 546.99 MPa (0.4% Y addition)→ 879.25 MPa (80% hot rolling) →1025.25 MPa (60% hot rolling + 60% cold rolling). The elongation of the alloy increases from 3.05% in the as-received state to 8.38% after dual-step rolling deformation with the similar trend as tensile fracture strength. The maximum superelastic strain increases with the addition of the Y element, but under the same strain, the as-received CuAlMn alloy exhibits higher superelasticity. Furthermore, the superelastic strain rapidly decreases after undergoing rolling deformation. In end, the microstructural origins of the improved mechanical properties were discussed in detail.-
Key words:
- CuAlMn alloy /
- dual-step rolling /
- microstructure /
- mechanical property /
- rare earth Y addition
-
图 4 Cu-11.36Al-5Mn-0.4Y合金TEM和SEM微观图像:(a) 明场图;(b) (a)中蓝色虚线框的选取电子衍射;(c) 析出相的明场图;(d) (c)的元素分布;(e) (c)中黄色虚线框的选取电子衍射;(f) 析出相的点扫结果
Figure 4. TEM and SEM microstructures of Cu-11.36Al-5Mn-0.4Y alloy: (a) bright-field image; (b) corresponding selected area electron diffraction (SAED) pattern of area in blue dashed box in (a); (c) bright-field image of precipitate phase; (d) elemental distribution of (c); (e) corresponding SAED pattern of area in yellow dashed box in (c); (f) result of EDS point scanning of precipitate phase
图 8 60% HR Cu-11.36Al-5Mn-0.4Y 合金TEM微观图像:(a) 高分辨图像,插图为黄色区域的放大图;(b) (a)图的FFT衍射图;(c) 低倍率明场图;(d) 位错明场图
Figure 8. TEM microstructures of 60% HR Cu-11.36Al-5Mn-0.4Y alloy: (a) high-resolution image, the inset shows the high magnification image of area in yellow dashed box; (b) fast Fourier transform (FFT) pattern of (a); (c) bright-field image; (d) bright-field image of dislocations
图 13 60%HR-60%CR Cu-11.36Al-5Mn-0.4Y合金TEM微观图像:(a) 明场图;(b) (a)中蓝色虚线框的分辨图像;(c) (b)的FFT衍射图;(d) (b)的IFFT图;(e/f) 位错的明场图;(g/h) 析出相的STEM图和元素分布,插图为A和B区域的选取电子衍射
Figure 13. TEM microstructures of 60% HR and 60% CR Cu-11.36Al-5Mn-0.4Y alloy: (a) bright-field image; (b) high-resolution image of area in blue dashed box in (a); (c) FFT pattern of (b); (d) inverse fast Fourier transform (IFFT) pattern of (b); (e/f) bright-field image of dislocations; (g/h) STEM image and elemental distribution of precipitate phase, inset shows the SAED pattern of area A and B
-
[1] ALANEME K K, ANAELE J U, BODUNRIN M O. Hot deformation processing of shape memory alloys: A review of effects on plastic flow behaviour, deformation mechanisms, and functional characteristics[J]. Alexandria Engineering Journal, 2022, 61(12): 12759-12783. doi: 10.1016/j.aej.2022.06.055 [2] RASHIDI K, SULONG A B, MUHAMAD N, et al. Martensitic transformation characteristics, mechanical properties and damping behavior of Cu–Al–Ni shape memory alloys: A review of their modifications and improvements[J]. Journal of Materials Research and Technology, 2024, 29: 2732-2749. doi: 10.1016/j.jmrt.2024.02.012 [3] LIU J L, HUANG H Y, XIE J X. Superelastic anisotropy characteristics of columnar-grained Cu–Al–Mn shape memory alloys and its potential applications[J]. Materials & Design, 2015, 85: 211-220. [4] WANG H Y, HUANG J L, XU D, et al. Comparison of microstructure and shape memory properties between two Cu-Al-Mn alloys produced by additive manufacturing technology[J]. Materialia, 2022, 26: 101594. doi: 10.1016/j.mtla.2022.101594 [5] LIU J L, CHEN Z H, HUANG H Y, et al. Microstructure and superelasticity control by rolling and heat treatment in columnar-grained Cu-Al-Mn shape memory alloy[J]. Materials Science and Engineering A, 2017, 696: 315-322. doi: 10.1016/j.msea.2017.04.085 [6] LI X H, LIAN H K, ZHANG Z W, et al. Columnar grain growth of superelastic CuAlMn alloy during directional recrystallization[J]. Materials Characterization, 2023, 201: 112943. doi: 10.1016/j.matchar.2023.112943 [7] AGRAWAL A, DUBE R K. Methods of fabricating Cu-Al-Ni shape memory alloys[J]. Journal of Alloys and Compounds, 2018, 750: 235-247. doi: 10.1016/j.jallcom.2018.03.390 [8] KUMAR P, LAGOUDAS D C. Introduction to Shape Memory Alloys[J]. Advanced Topics of Thin-Walled Structures, 2021. [9] ALANEME K K, ANAELE J U, OKOTETE E A. Martensite aging phenomena in Cu-based alloys: Effects on structural transformation, mechanical and shape memory properties: A critical review[J]. Scientific African, 2021, 12: e760. [10] ALANEME K K, OKOTETE E A. Reconciling viability and cost-effective shape memory alloy options–A review of copper and iron based shape memory metallic systems[J]. Engineering Science and Technology An International Journal, 2016, 19(3): 1582-1592. doi: 10.1016/j.jestch.2016.05.010 [11] DAWOOD N, ALI A. Effect of Aging on Corrosion Behavior of Martensite Phase in Cu-Al-Ni Shape Memory Alloy[J]. Key Engineering Materials, 2022, 911: 96-102. doi: 10.4028/p-3jm065 [12] MUNTASIR BILLAH A, RAHMAN J, ZHANG Qi. Shape memory alloys (SMAs) for resilient bridges: A state-of-the-art review[J]. Structures, 2022, 37: 514-527. doi: 10.1016/j.istruc.2022.01.034 [13] XI X Y, TANG H, ZHANG J T, et al. Unraveling effect of Nb addition on the microstructure, phase transformation behaviour and shape memory effect of CuAlMn alloy fabricated by laser powder bed fusion[J]. Journal of Materials Research and Technology, 2024, 29: 4163-4172. doi: 10.1016/j.jmrt.2024.02.147 [14] TU J, WANG Y N, HE D Y, et al. Ductile CuAlMn shape memory alloys with higher strength by Fe alloying and grain boundary engineering[J]. Materials Science and Engineering A, 2022, 841: 143032. doi: 10.1016/j.msea.2022.143032 [15] SUN L, HUANG W M, DING Z M, et al. Stimulus-responsive shape memory materials: A review[J]. Materials & Design, 2012, 33: 577-640. [16] JIAO Z X, WANG Q Z, YIN F X, et al. Novel laminated multi-layer graphene/Cu–Al–Mn composites with ultrahigh damping capacity and superior tensile mechanical properties[J]. Carbon, 2022, 188: 45-58. doi: 10.1016/j.carbon.2021.11.055 [17] SUTOU Y, OMORI T, YAMAUCHI K, et al. Effect of grain size and texture on pseudoelasticity in Cu–Al–Mn-based shape memory wire[J]. Acta Materialia, 2005, 53(15): 4121-4133. doi: 10.1016/j.actamat.2005.05.013 [18] LIU N, LI Z, XU G Y, et al. Effect of tellurium on machinability and mechanical property of CuAlMnZn shape memory alloy[J]. Materials Science and Engineering A, 2011, 528(27): 7956-7961. doi: 10.1016/j.msea.2011.07.030 [19] LU X L, CHEN F, LI W S, et al. Effect of Ce addition on the microstructure and damping properties of Cu–Al–Mn shape memory alloys[J]. Journal of Alloys and Compounds, 2009, 480(2): 608-611. doi: 10.1016/j.jallcom.2009.01.134 [20] JIAO Z X, YIN F X, WANG Q Z, et al. Refining effect of an intermetallic inoculant on a Cu–Al–Mn shape memory alloy[J]. Materials Chemistry and Physics, 2022, 280: 125835. doi: 10.1016/j.matchemphys.2022.125835 [21] JIAO Z X, WANG Q Z, YIN F X, et al. Effects of Cu51Zr14 inoculant and caliber rolling on microstructures and comprehensive properties of a Cu–Al–Mn shape memory alloy[J]. Materials Science and Engineering A, 2020, 772: 138773. doi: 10.1016/j.msea.2019.138773 [22] ĆORIĆ D, ŽMAK I. Influence of Ausforming Treatment on Super Elasticity of Cu-Zn-Al Shape Memory Alloy for Seismic Energy Dissipaters[J]. Buildings, 2021, 11(1): 22. doi: 10.3390/buildings11010022 [23] 千佳祥, 杨院霞, 张江, 等. 稀土Y元素对CuAlMn合金晶粒细化及其力学性能的影响[J]. 材料工程, 2023, 52(8): 214-224.QIAN Jiaxiang, YANG Yuanxia, ZHANG Jiang, et al. Effect of rare earth Y element on grain refinement and mechanical property of CuAlMn alloy[J]. Journal of Materials Engineering, 2023, 52(8): 214-224(in Chinese). [24] LA ROCA P, ISOLA L, VERMAUT P, et al. β-grainsize Effects on the 18R-martensite Microstructure in Cu-based SMA[J]. Procedia Materials Science, 2015, 8: 1133-1139. doi: 10.1016/j.mspro.2015.04.177 [25] LA ROCA P, ISOLA L, VERMAUT P, et al. Relationship between martensitic plate size and austenitic grain size in martensitic transformations[J]. Applied physics letters, 2015, 106(22). [26] MORRISON K R, CHERUKARA M J, KIM H, et al. Role of grain size on the martensitic transformation and ultra-fast superelasticity in shape memory alloys[J]. Acta Materialia, 2015, 95: 37-43. doi: 10.1016/j.actamat.2015.05.015 [27] ZHANG Y K, XU L Y, ZHAO L, et al. Process-microstructure-properties of CuAlNi shape memory alloys fabricated by laser powder bed fusion[J]. Journal of Materials Science & Technology, 2023, 152: 1-15. [28] KRACHAN T, STEL MAKHOVYCH B, KUZ MA Y. The Y–Cu–Al system[J]. Journal of alloys and compounds, 2003, 349(1): 134-139. [29] MANDAL G K, STANFORD N, HODGSON P, et al. Static recrystallisation study of as-cast austenitic stainless steel[J]. Materials Science and Engineering A, 2013, 576: 118-125. doi: 10.1016/j.msea.2013.03.076 [30] MALLIK U S, SAMPATH V. Influence of quaternary alloying additions on transformation temperatures and shape memory properties of Cu–Al–Mn shape memory alloy[J]. Journal of Alloys and Compounds, 2009, 469(1-2): 156-163. doi: 10.1016/j.jallcom.2008.01.128 [31] YE C, TELANG A, GILL A S, et al. Gradient nanostructure and residual stresses induced by Ultrasonic Nano-crystal Surface Modification in 304 austenitic stainless steel for high strength and high ductility[J]. Materials Science and Engineering A, 2014, 613: 274-288. doi: 10.1016/j.msea.2014.06.114 [32] ZHANG Y K, XU L Y, ZHAO L, et al. Deformation mechanism of Cu-Al-Ni shape memory alloys fabricated via laser powder bed fusion: Tension-compression asymmetry[J]. Journal of Materials Science & Technology, 2023, 167: 14-26. [33] XIAO Z, FANG M, LI Z, et al. Structure and properties of ductile CuAlMn shape memory alloy synthesized by mechanical alloying and powder metallurgy[J]. Materials & Design, 2014, 58: 451-456. [34] OMORI T, KUSAMA T, KAWATA S, et al. Abnormal Grain Growth Induced by Cyclic Heat Treatment[J]. Science, 2013, 341(6153): 1500-1502. doi: 10.1126/science.1238017 [35] ZENG M T, YANG Y, TAN Y B, et al. Recrystallization behavior and texture evolution of cryo-rolled GH159 superalloy with an ultra-high strength[J]. Materials Characterization, 2023, 197: 112656. doi: 10.1016/j.matchar.2023.112656 [36] YANG W G, LI M G, ZHOU T, et al. Deformation behavior and dynamic recrystallization mechanism of a novel high Nb containing TiAl alloy in (α+γ) dual-phase field[J]. Journal of Alloys and Compounds, 2023, 945: 169250. doi: 10.1016/j.jallcom.2023.169250 [37] LIU J W, ZHANG K, YANG Y, et al. Grain boundary α-phase precipitation and coarsening: Comparing laser powder bed fusion with as-cast Ti-6Al-4V[J]. Scripta Materialia, 2022, 207: 114261. doi: 10.1016/j.scriptamat.2021.114261 [38] MAZZER E M, KIMINAMI C S, BOLFARINI C, et al. Phase transformation and shape memory effect of a Cu-Al-Ni-Mn-Nb high temperature shape memory alloy[J]. Materials Science and Engineering A, 2016, 663: 64-68. doi: 10.1016/j.msea.2016.03.017 [39] JOHN H, GRRGORY S ROHRER, ANTHONY R. Recrystallization and related annealing phenomena (Third Edition), Chapter 2-The Deformed State[M]. Oxford: Elsevier, 2017, 13-79. [40] WANG L, QIAO J W, MA S G, et al. Mechanical response and deformation behavior of Al0.6CoCrFeNi high-entropy alloys upon dynamic loading[J]. Materials Science and Engineering A, 2018, 727: 208-213. doi: 10.1016/j.msea.2018.05.001 [41] 李杰, 吴凯迪, 牛利冲, 等. 退火温度对(Fe50Mn30Co10Cr10)97Al3高熵合金再结晶行为及力学性能的影响[J]. 稀有金属材料与工程, 2023, 52(12): 4251-4259.LI Jie, WU Kaidi, NIU Lichong, et al. Effect of annealing temperatures on recrystallization behavior and mechanical properties of (Fe50Mn30Co10Cr10)97Al3 High-Entropy Alloy[J]. Rare Metal Materials and Engineering, 2023, 52(12): 4251-4259(in Chinese). [42] ZHANG Y T, WEI D X, CHEN Y, et al. Non-negligible role of gradient porous structure in superelasticity deterioration and improvement of NiTi shape memory alloys[J]. Journal of Materials Science & Technology, 2024, 186: 48-63. [43] SIMON T, KRӦGER A, SOMSEN C, et al. On the multiplication of dislocations during martensitic transformations in NiTi shape memory alloys[J]. Acta Materialia, 2010, 58(5): 1850-1860. doi: 10.1016/j.actamat.2009.11.028 [44] WANG H S, FAN M Y, CUI Y, et al. Effects of Cr on the abnormal grain growth of Cu-Al-Mn-Ni-Cr superelastic alloy[J]. Materials Today Communications, 2024, 38: 108432. doi: 10.1016/j.mtcomm.2024.108432 [45] KUMAR A, PALANI I A, YADAV M. Comprehensive study of microstructure, phase transformations, and mechanical properties of nitinol alloys made of shape memory and superelastic wires and a novel approach to manufacture Belleville spring using wire arc additive manufacturing[J]. Materials Today Communications, 2024, 38: 107881. doi: 10.1016/j.mtcomm.2023.107881 [46] ZHANG M G, LI X Z, WANG B L, et al. Prominent superelastic response induced by Ni4Ti3 phase in NiTi alloys fabricated via wire-arc directed energy deposition[J]. Materials Science and Engineering A, 2024, 897: 146366. doi: 10.1016/j.msea.2024.146366 -
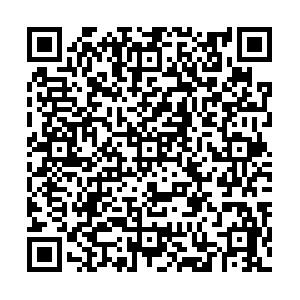
计量
- 文章访问数: 49
- HTML全文浏览量: 33
- 被引次数: 0