Coal tar pitch pitch modification of the SiO interface enhances the cycling stability of lithium-ion batteries
-
摘要: 煤焦油沥青具有高碳含量、可调控性和经济性等优点,在制备碳材料和碳复合材料中具有广泛的应用前景。采用沥青对一氧化硅界面改性是抑制一氧化硅自身的膨胀、提升首次库伦效率和循环稳定性的有效策略。为改善沥青与基体材料间的结合性能,本研究采用添加黏结剂的方式制备负极材料。首先,通过空气交联低温条件下制备高软化点、高结焦值的改性沥青。以聚乙烯吡咯烷酮(PVP)为黏结剂,浸润结合一氧化硅和改性沥青,制备得到前驱体(SiO@PVP@pitch)。通过原位聚合,将改性沥青转化为中间相沥青,然后高温炭化得到SiO/C复合材料,使碳涂层有一定的机械强度又具备导电性。偏光显微镜结果表明碳涂层良好的流线型结构,纤维更加细腻。高分辨透射电子显微镜( HRTEM )结果表明,SiO表面存在厚度约为90 - 100 nm的含有石墨晶格的碳包覆层。SiO和改性沥青按照5∶3比例下在400 ℃和900 ℃的温度条件下聚合和炭化,复合材料表现出优异的电化学性能,在0.5 A/g电流密度下具有550 mA·h/g的高比容量,可逆比容量660 mA·h/g,200次循环后比容量保持率为83.33 %,在1.5 A/g电流密度下的比容量为472.8 mA·h/g。电化学阻抗谱( EIS )结果也证明了碳包覆层可以有效提高复合材料的导电性,从而增强SiO电极的循环稳定性。Abstract: Coal tar pitch has the advantages of high carbon content, controllability and economy, and broad application prospects in preparing carbon materials and carbon composites. Using pitch to modify the interface of silicon monoxide is an effective strategy to inhibit the expansion of silicon monoxide itself and improve the first Coulomb efficiency and cycle stability. To improve the bonding performance between pitch and matrix materials, this study prepared anode materials by adding binders. Firstly, we prepared a modified pitch with a high softening point, high coking value, and good rheology by air crosslinking at low temperatures. The precursor (SiO@PVP@pitch) was prepared b, using polyvinylpyrrolidone (PVP) as a binder and infiltrating combined with silicon monoxide and modified pitch. Through in-situ polymerization, the modified pitch is converted into mesophase pitch and then carbonized at high temperature to obtain SiO/C composites, so that the carbon coating has certain mechanical strength and electrical conductivity. The results of the polarizing microscope show that the carbon coating has a good streamlined structure and the fiber is more delicate. High-resolution transmission electron microscopy (HRTEM) results show that there is a carbon coating layer containing graphite lattice with a thickness of about 90-100 nm on the surface of SiO. The synthesized SiO/C - 5∶3 - 4 h composites showed excellent performance. It has a high specific capacity of 550 mA·h/g at 0.5 A/g, a reversible capacity of 660 mA·h/g, and a capacity retention rate of 83.33 % after 200 cycles. The capacity is 472.8 mA·h/g at 1.5 A/g. Electrochemical impedance spectroscopy (EIS) results also prove that the carbon coating layer can effectively improve the conductivity of the composite material, thereby enhancing the cycle stability of the SiO electrode.
-
Key words:
- anode /
- core-shell structure /
- mesophase pitch /
- carbon coating /
- lithium-ion battery
-
图 5 SiO/C - 5∶3 - 4 h的高分辨透射电镜(HRTEM)图像(a)、(b)和(c)为不同放大倍数下的高分辨透射电镜(HRTEM)图像(d)、(e)和(f)为碳涂层的厚度 (g) TEM - Mapping图像
Figure 5. High-resolution transmission electron microscopy (HRTEM) images (a), (b), and (c) are high-resolution transmission electron microscopy (HRTEM) images at different magnifications (d), (e) and (f) are the thickness of the carbon coating (g) TEM-Mapping images of SiO/C - 5∶3 - 4 h
图 8 复合材料电化学性能图 (a)-(b) SiO/C - 5∶3 - 4 h复合材料和SiO CV曲线,电压范围为0.01 - 1.8 V,扫描速率为0.1 mV·s−1;(c) - (d) SiO/C - 5∶3 - 4 h复合材料和SiO在第1个、第2个、第3个、第100个和第200个循环的充放电电压曲线。
Figure 8. Electrochemical performance of SiO/C-5∶3-4 h composite material. (a) CV curve of SiO/C - 5∶3 - 4 h composite material, the voltage range is 0.01-1.8 V, and the scanning rate is 0.1 mV·s−1. The charge and discharge voltage curves of (c) - (d) SiO/C - 5∶3 - 4 h composites and SiO at the 1st, 2 nd, 3 rd, 100 th and 200 th cycles.
图 11 奈奎斯特图和相应的等效电路模型EIS图谱(a) SiO/C复合材料阻抗(b) SiO循环前后阻抗(c) SiO/C - 5∶3 - 4 h循环前后阻抗
Figure 11. Nyquist diagram and EIS diagram of the corresponding equivalent circuit model (a) Impedance of SiO/C composites (b) Impedance before and after SiO cycling (c) Impedance before and after SiO/C - 5∶3 - 4 h cycling
图 12 SiO和SiO/C - 5∶3 - 4 h复合材料循环前和200个循环后的电极的体积变化的SEM图像(a) SiO循环前(b) SiO循环后(c) SiO/C - 5∶3 - 4 h循环前(d) SiO/C - 5∶3 - 4 h循环后
Figure 12. SEM images of volume change of SiO and SiO/C-5∶3 - 4 h composite electrode before and after 200 cycles (a) before SiO cycle (b) after SiO cycle (c) before SiO/C-5∶3 - 4 h cycle (d) after SiO/C-5∶3 - 4 h cycle
表 1 改性沥青的制备条件
Table 1. Preparation conditions of modified pitch
Air purge air volume/(L min−1) soaking time/h The heating rate of different temperature stages/(℃min−1) 25℃ - 100℃ 100℃- 290℃ 290℃ - 320℃ 1 10 5 3 1 表 2 原料沥青与改性煤沥青的特性和元素分析对比表
Table 2. Characteristics and elemental analysis comparison table of raw pitch and modified coal tar pitch
Sp/℃ CV/% pitch analysis/% elementary analysis/% HS HI-TS TI-QS QI N C H S O Pitch 31 32 30.98 53.03 18.55 0.031 1.145 92.474 4.979 0.356 1.046 Modified pitch 240 80 10.77 12.37 63.85 13 1.21 92.671 4.303 0.145 2.04 Note:(1) Organic element analysis was based on air drying, and O content was calculated by subtraction method.(2) Softening point (SP) was measured by thermal mechanical analyzer;(3) Coking value (CV) was determined by ' GB/T 8727-2008 determination method of the coking value of coal tar pitch products ';(4) HS and HI-TS were measured by soxhlet extraction, where HS is n-hexane soluble, HI-TS is n-hexane insoluble-toluene soluble;(5) TI-QS is toluene insoluble-quinoline soluble, its content is the percentage minus HS, HI-TS, QI content;(6) QI content is determined by the national standard GB/T 2293-2019 quinoline insoluble test method for coking pitch products. 表 3 SiO/C复合材料中SiO和pitch的百分比及炭化条件
Table 3. Percentages of SiO and pitch in SiO/C composites and carbonization conditions
composites materials pitch:SiO SiO content pitch content Polymerization Temperature/℃ SiO/C -5∶3 - 4 h 5∶3 37.5 % 62.5 % 400℃-4 h 900℃ - 3 h SiO/C -5∶2 - 4 h 5∶2 28.5 % 71.5 % 400℃-4 h 900℃ - 3 h SiO/C - 5∶4 - 4 h 5∶4 44.4 % 55.56 % 400℃-4 h 900℃ - 3 h SiO/C -5∶3 - 0 h 5∶3 37.5 % 62.5 % 400℃-900℃ - 3 h -
[1] ESCAMILLA-PÉREZ A M, AUDE R, SOPHIE G, et al. Pitch-based carbon/nano-silicon composite, an efficient anode for Li-ion batteries[J]. RSC Advances, 2019, 9(19): 10546-10553. doi: 10.1039/C9RA00437H [2] HUANG Ben, CHU Binbin, HUANG Tao, et al. Nitrogen-Doped Carbon-Coating Disproportionated SiO Materials as Long Cycling Stable Anode for Lithium-Ion Batteries[J]. Molecules, 2021, 26(6): 1536. doi: 10.3390/molecules26061536 [3] SUN Wenhao, SUN Xiaogang, PENG Qifan, et al. Nano-MgO/AB decorated separator to suppress shuttle effect of lithium-sulfur battery[J]. Nanoscale Advances, 2019, 1(4): 1589-1597. doi: 10.1039/C8NA00420J [4] GUO Chenfeng, MAN Jingxuan, and WANG Dianlong. Nitrogen-doped carbon-coated SiO nanoparticles Co-modified with nitrogen-doped graphene as a superior anode material for lithium-ion batteries[J]. RSC Adv, 2014, 4(67): 35717-35725. doi: 10.1039/C4RA06678B [5] YU B C, YOON H, KIM J H, et al. A New Approach to Synthesis of Porous SiOx Anode for Li-ion Batteries via Chemical Etching of Si Crystallites[J]. Electrochimica Acta, 2014, 117: 426-430. doi: 10.1016/j.electacta.2013.11.158 [6] . YU B C, YOON H, PARK C M, et al. "Reaction mechanism and enhancement of cyclability of SiO anodes by surface etching with NaOH for Li-ion batteries[J]. " Journal of Materials Chemistry A 1.15(2013): 4820. [7] . YOON H, PARK C M and SOHN, Modified SiO as a high-performance anode for Li-ion batteries[J]. Journal of Power Sources, 2013, 222: 129-134. [8] DOH C H, PARK C W, SHIN H M, et al. A new SiO/C anode composition for lithium-ion battery[J]. Journal of Power Sources, 2008, 179(1): 367-370. doi: 10.1016/j.jpowsour.2007.12.074 [9] . ZHANG Xiangwu, JI Liwen, TOPRAKCI O, et al. Electrospun Nanofiber-Based Anodes, Cathodes, and Separators for Advanced Lithium-Ion Batteries[J]. Polymer reviews, 2011, 51(3): 239-264: 239-264. [10] . GUO Juchen, YANG Zichao and ARCHER L A, Mesoporous silicon@carbon composites via nanoparticle-seeded dispersion polymerization and their application as lithium-ion battery anode materials[J]. Journal of Materials Chemistry A, 2013, 1(18): 5709. [11] JEONG J H, KIM K H, JUNG D W, et al. High-performance characteristics of silicon inverse opal synthesized by the simple magnesium reduction as anodes for lithium-ion batteries[J]. Journal of Power Sources, 2015, 300: 182-189. doi: 10.1016/j.jpowsour.2015.09.064 [12] . CHEN Bingjie, CHEN Lu, FENG Yutong, et al. Zero-strain high-capacity Silicon/Carbon Anode Enabled by a MOF-Derived Space-Confined Single-Atom Catalytic Strategy for Lithium-Ion Batteries[J]. Advanced Materials, 2022, 34(21). [13] RU Ziwei, ZHANG Xin, ZHANG Man, et al. Bimetallic-MOF-Derived ZnxCo3-xO4/Carbon Nanofiber Composited Sorbents for High-Temperature Coal Gas Desulfurization[J]. Environmental Science & Technology, 2022, 56(23): 17288-17297. [14] MAJEED M K, MA Guangyao, CAO Yanxiu, et al. Metal-Organic Frameworks-Derived Mesoporous Si/SiOx@NC Nanospheres as a Long-Lifespan Anode Material for Lithium-Ion Batteries[J]. Chemistry-A European Journal, 2019, 25(51): 11991-11997. doi: 10.1002/chem.201903043 [15] YANG Hongxun, XIE Yue, ZHU Miaomiao, et al. Hierarchical porous MnCo2O4 yolk-shell micro-spheres from MOFs as secondary nanomaterials for high-power lithium-ion batteries[J]. Dalton Transactions, 2019, 48: 9205-9213. doi: 10.1039/C9DT00613C [16] MASTROPIETRO T F. Metal-organic frameworks and plastic: an emerging synergic partnership[J]. Science and technology of advanced materials, 2023, 24(1): 2189890-2189890. doi: 10.1080/14686996.2023.2189890 [17] LIN Yangfan, CHEN Yifan, ZHANG Yaguang, et al. Wet-chemical synthesized MCMB@Si@C microspheres for high-performance lithium-ion battery anodes[J]. Chemical Communications (Cambridge, England), 2018, 54(68): 9466-9469. doi: 10.1039/C8CC04797A [18] CHENG Xianglin, ZHAQ ingfang, LI Xuejun, et al. Modified characteristics of mesophase pitch prepared from coal tar pitch by adding waste polystyrene[J]. Fuel Processing Technology, 2008, 89(12): 1436-1441. doi: 10.1016/j.fuproc.2008.07.003 [19] LIN J H, KO T H, KUO W S, et al. Mesophase Pitch Carbon Coated with Phenolic Resin for the Anode of Lithium-Ion Batteries[J]. Energy & Fuels, 2010, 24(7): 4090-4094. [20] HUANG Zhihao, DANG Guoju, JIANG Wenping, et al. A Low-Cost and Scalable Carbon Coated SiO-Based Anode Material for Lithium-Ion Batteries[J]. ChemistryOpen, 2021, 10(3): 380-386. doi: 10.1002/open.202000341 [21] SIRIWACHIRACHAL C and PONGJANYAKUL T. Particle Agglomeration of Acid-Modified Tapioca Starches: Characterization and Use as Direct Compression Fillers in Tablets[J]. Pharmaceutics, 2022, 14(6): 1245. doi: 10.3390/pharmaceutics14061245 [22] MAMIYA M, KIKUCHI M, TAKEI H. Crystallization of fine silicon particles from silicon monoxide[J]. Journal of Crystal Growth, 2002, 237-239: 1909-1914. doi: 10.1016/S0022-0248(01)02244-8 [23] LI Dechang, XU Wanfei, CHENG Huiyuan, et al. One-Step Thermochemical Conversion of Biomass Waste into Superhydrophobic Carbon Material by Catalytic Pyrolysis[J]. Global Challenges, 2020, 4(4): 1900085. doi: 10.1002/gch2.201900085 [24] MORISSET A, FAMPROKIS T, HAUG F J, et al. In Situ Reflectometry and Diffraction Investigation of the Multiscale Structure of p-Type Polysilicon Passivating Contacts for c-Si Solar Cells[J]. ACS Applied Materials & Interfaces, 2022, 14(14): 16413-16423. [25] . QIAN Guanghan, RAHMAN S A, GOH B T. Controlled growth of Si-based heterostructure nanowires and their structural and electrical properties[J]. Nanoscale Research Letters, 2015, 10(1). [26] NETOR D E T, DAVID H L, FRANCISCO J F J, JOSE A L L, et al. Evaluation of optical and electronic properties of silicon nano-agglomerates embedded in SRO: applying density functional theory[J]. Nanoscale research letters, 2014, 9(1): 507-507. doi: 10.1186/1556-276X-9-507 [27] LEE G, SCHWEIZER S L, WEHRSPOHN R B. Wehrspohn, CMOS-compatible metal-stabilized nanostructured Si as anodes for lithium-ion micro batteries[J]. Nanoscale research letters, 2014, 9(1): 613-613. doi: 10.1186/1556-276X-9-613 [28] SHANG Huishan, PAN Kecheng, ZHANG Lu, et al. , Enhanced Activity of Supported Ni Catalysts Promoted by Pt for Rapid Reduction of Aromatic Nitro Compounds[J]. Nanomaterials, 2016, 6(6): 103. doi: 10.3390/nano6060103 [29] JEONG S Y, CHO J S. Porous Hybrid Nanofibers Comprising ZnSe/CoSe₂/Carbon with Uniformly Distributed Pores as Anodes for High-Performance Sodium-Ion Batteries[J]. Nanomaterials, 2019, 9(10): 1362. doi: 10.3390/nano9101362 [30] HINSTSHO N, SHAIKJEE A, MASENDA H, et al. Direct synthesis of carbon nanofibers from South African coal fly ash[J]. Nanoscale research letters, 2014, 9(1): 387-387. doi: 10.1186/1556-276X-9-387 [31] LEE S E, KIM J H, LEE Y S, et al. Effect of coke orientation on the electrochemical properties of lithium-ion battery anode[J]. Journal of Applied Electrochemistry, 2021, 51(10): 1407-1418. doi: 10.1007/s10800-021-01581-x [32] MOCHIDA S, FEI Youqing, OYAMA T, et al. Carbonization of coal-tar pitch into lump needle coke in a tube bomb[J]. Journal of Materials Science, 1987, 22(11): 3989-3994. doi: 10.1007/BF01133349 [33] LI Dechang, XU Wanfei, CHENG Huiyuan, XI Kunfang, et al. One-Step Thermochemical Conversion of Biomass Waste into Superhydrophobic Carbon Material by Catalytic Pyrolysis[J]. Glob Chall, 2020, 4(4): 1900085. doi: 10.1002/gch2.201900085 [34] PENG Guiming, ELLIS J E, XU Xueqing, et al. In Situ Grown TiO2 Nano-spindles Facilitate the Formation of Holey Reduced Graphene Oxide by Photodegradation[J]. ACS Applied Materials & Interfaces, 2016, 8(11): 7403-7410. [35] . GUO Jiangyi, ZHANG Weilin. CHEN Datong, et al. Control over Electrochemical CO2 Reduction Selectivity by Coordination Engineering of Tin Single-Atom Catalysts[J]. Advanced Science, 2021, 8(23). [36] WANG Bing, HE Quanguo, LI Guangli, et al. Sensitive Determination of Trace 4-Nitrophenol in Ambient Environment Using a Glassy Carbon Electrode Modified with Formamide-Converted Nitrogen-Doped Carbon Materials[J]. International Journal of Molecular Sciences, 2022, 23(20): 12182. doi: 10.3390/ijms232012182 [37] JEONG M G, ISLAM M, DU H L, et al. Nitrogen-doped Carbon Coated Porous Silicon as High-Performance Anode Material for Lithium-Ion Batteries[J]. Electrochimica Acta, 2016, 209: 299-307. doi: 10.1016/j.electacta.2016.05.080 [38] ZHENG, Zhiming, ZAO Yi, ZHANG Qiaobao, et al. Robust erythrocyte-like Fe2O3@carbon with yolk-shell structures as high-performance anode for lithium-ion batteries[J]. Chemical Engineering Journal, 2018, 347: 563-573. doi: 10.1016/j.cej.2018.04.119 [39] LI Qiongguang, WANG Yanhong, LU Bin, et al. Hollow core-shell structured Si@NiAl-LDH composite as high-performance anode material in lithium-ion batteries[J]. Electrochimica Acta, 2020, 331: 135331. doi: 10.1016/j.electacta.2019.135331 [40] 石永刚, 张志勇, 陈彬, 等. 硅化镁还原CO2一步原位合成Si/C复合负极[J]. 复合材料学报. 2021, 38(10): 3522.SHENG Yonggang, ZHANG Zhiyong, CHEN Bin, et al. In-situ synthesis of Si/C composites anode by one-step reduction of CO2 with magnesium silicide[J]. Acta Materiae Compositae Sinica, 2021, 38(10): 3522. (in Chinese). -
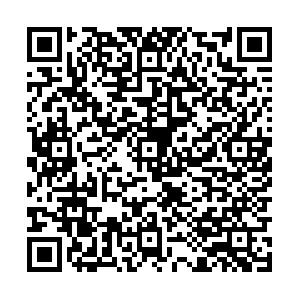
计量
- 文章访问数: 81
- HTML全文浏览量: 73
- 被引次数: 0