Bearing and failure characteristics of conformal antenna sandwich structure under edge compression
-
摘要: 共形天线由于能够实现电磁-承载-轻量化的结构功能一体化设计,因此相较于传统机载天线,共形天线更加适应现代化发展趋势,而复合材料夹层结构作为多材质叠层材料,层间承载能力较弱,天线的埋设又会引入新的界面,会对原有结构的传载特性和结构破坏模式产生重大影响,另外结构的制备技术和成型工艺发生改变也会影响力学性能。本文针对一种共形天线夹层结构,通过试验和有限元方法分析了该结构在侧压载荷下的承载特性和失效机制,并与复合材料泡沫夹层结构进行比较。结果表明,天线界面的引入使结构质量增加了38.87%,结构比强度提高1.35%,比刚度下降21.72%。对于结构的失效模式,天线阵列的引入导致结构失效模式从以分层渐进失效为主的中部折断转为沿夹具夹持端应力集中处扩展的基体压缩渐进失效;同时,共形天线夹层结构加工工艺存在溢胶问题,溢胶程度的不可控性会导致结构承载和破坏机制的变化,因此控制溢胶对提高结构力学性能至关重要。Abstract: Conformal antennas, due to their capability of integrating electromagnetic functionality, structural support, and lightweight design, exhibit greater adaptability to modern development trends compared to conventional airborne antennas. However, sandwich structures utilizing composite materials with multiple layers and materials possess inherently weak interlayer load-bearing capabilities. Embedding antennas within these structures significantly alters their load-carrying characteristics and structural failure modes due to the introduction of new interfaces. Furthermore, uncertainties arise regarding whether the structure can fulfill design requirements following changes in manufacturing technology and forming process. This paper focused on the bearing characteristics and failure mechanisms of a conformal antenna sandwich structure (CASS) under lateral pressure loads, employing both experimental and finite element analysis methodologies. We benchmarked our findings against a composite foam sandwich structure (CFSS) for comparison. Our results reveal that incorporating the antenna interface increases the structural weight by 38.87%, enhances the specific strength by 1.35%, but diminishes the specific stiffness by 21.72%. Notably, the introduction of the antenna array shifts the structural failure mode from a central fracture dominated by layered progressive failure to a progressive matrix compression failure extending along stress concentrations at the clamping ends of the fixture. Additionally, the CASS processing technology encounters glue overflow issues, and the uncontrollable degree of overflow can modify the load-bearing and failure mechanisms of the structure. Thus, mitigating glue overflow becomes pivotal for optimizing the mechanical properties of the CASS.
-
表 1 预浸料的力学性能参数
Table 1. Mechanical properties of prepreg
Property Value Units Description $\rho $ 1.8 ${\text{g/c}}{{\text{m}}^{\text{3}}}$ density ${E_1}$ 23500 MPa longitudinal modulus ${E_2}$ 22000 MPa transverse modulus ${E_3}$ 15000 MPa out-of-plane equivalent modulus ${\mu _{12}}$ 0.18 - poisson ratio ${\mu _{13}},{\mu _{23}}$ 0.2/0.18 - poisson ratio ${G_{12}}$ 3500 MPa shear modulus $ {G}_{13},{G}_{23} $ 4000 MPa shear modulus ${X_t}$ 600 MPa longitudinal tensile strength ${X_c}$ 450 MPa longitudinal compressive strength ${Y_t}$ 500 MPa transverse tensile strength ${Y_c}$ 420 MPa Transverse compressive strength ${Z_t}$ 59.7 MPa Out-of-plane tensile strength ${Z_c}$ 215 MPa Out-of-plane compressive strength ${S_{12}}$ 120 MPa longitudinal-transverse shear strength ${S_{13}} = {S_{23}}$ 70 MPa interlaminar shear strength 表 2 PMI泡沫的力学性能参数
Table 2. Mechanical properties of PMI foam
Property Value Units Description $\rho $ 71 ${\text{kg/}}{{\text{m}}^{\text{3}}}$ density $E$ 114.5 MPa young's modulus $\mu $ 0.375 - poisson ratio 表 3 胶膜的力学性能参数
Table 3. Mechanical properties of adhersive film
Property Value Units Description $\rho $ 1.16 $ {\text{g/c}}{{\text{m}}^{\text{3}}} $ density $S$ 18.7 MPa shearing strength ${E_{nn}} = {E_{ss}} = {E_{tt}}$ 2500 MPa elastic modulus ${K_{nn}} = {K_{ss}} = {K_{tt}}$ 1000 GPa initial stiffness $t_n^0$ 6.1 MPa outside shear strength $t_s^0 = t_t^0$ 30 MPa in-plane shear strength ${G_{{\rm I}C}}$ 0.243 N/mm I-type critical fracture energy ${G_{{\rm I}{\rm I}C}},{G_{{\rm I}{\rm I}{\rm I}C}}$ 0.514 N/mm Type II and Type III critical
fracture energy release rates表 4 CFSS与CASS的抗压强度与抗压刚度
Table 4. Compressive strength and stiffness of CFSS and CASS
Feature CFSS CASS Mass/g 265 368 Density/(g·m−3) 1.178 1.63 compressive strength/MPa 87.38 122.98 Test value of equivalent compressive
stiffness/kN9371 10183 Theoretical prediction value of equivalent compressive stiffness/kN 11313 12134 -
[1] 史则颖, 叶冬, 彭子寒等. 飞行器共形天线新型制造工艺及应用研究进展[J]. 航空学报, 2021, 42(10): 157-173.SHI Z, YE D, PENG Z, et al. Research progress on novel manufacturing approaches of conformal antenna for aircraft[J]. Acta Aeronautica et Astronautica Sinica, 2021, 42(10): 157-173(in Chinese). [2] 杨波, 赵培林, 蔡三军等. 新一代战斗机座舱盖关键技术与设计方案[J]. 航空学报, 2020, 41(6): 307-317.YANG B, ZHAO P, CAI S, et al. Key technologies and design of new generation fighter canopy[J]. Acta Aeronautica et Astronautica Sinica, 2020, 41(6): 307-317(in Chinese). [3] AFRL成功验证SIXA结构件[J]. 军民两用技术与产品, 2007, 3: 35-35.AFRL successfully verified the SIXA structural component[J]. Dual Use Technologies & Products, 2007, 3: 35-35. (in Chinese) [4] 睿达. 共形天线与传感器飞机[J]. 运输机工程, 2008, 1: 2-7.RUI D. Conformal antenna and sensor aircraft[J]. Transport Engineering, 2008, 1: 2-7(in Chinese). [5] 吴波, 谈腾. 机载智能蒙皮天线技术的研究进展[J]军民两用技术与产品, 2018(7): 55-58+61.WU B, TAN T. Research progress in airborne intelligent skin antenna technology[J]. Dual-use Technology and Products for Military and Civil Use, 2018(7): 55-58+61. (in Chinese) [6] ZHAO W, XIE Z, ZHANG P, et al. Damage tolerance-based design of conformal antenna composite structure[J]. Mechanics of Advanced Materials and Structures, 2022, 29(26): 5530-5541. doi: 10.1080/15376494.2021.1958033 [7] NAZARI A R, KABIR M Z, HOSSEINI-TOUDESHKY H, et al. Investigation of progressive failure in the composite sandwich panels with elastomeric foam core under concentrated loading[J]. Journal of Sandwich Structures and Materials, 2019, 21(8): 2585-2615. doi: 10.1177/1099636217719424 [8] LEI H, ZHAO Z, GUO X, et al. Research progress on the design and manufacture technology of lightweight multifunctional spacecraft structures[J]. Aerospace Materials & Technology, 2021, 51(4): 10-22. [9] XIE Z, WU S, WANG W, et al. Mechanical tests on broadband conformal antenna structure[J]. Journal of Nanjing University of Aeronautics & Astronautics, 2015, 47(6): 811-17. [10] YIN B, XU X, GU X. Damage simulation and strength prediction of foam-core sandwich structure with and without antennas[J]. Journal of Materials Science and Engineering, 2013, 31(5): 708-714,727. [11] LI P, XU W Y. The diagnosis of manufacturing defects in composite material radome based on antenna's far field data[J]. Materials Research Innovations, 2015, 19: 277-282. [12] 夹层结构侧压性能试验方法. 国家标准, GB/T 1454-2021.Test method for lateral compression performance of sandwich structures. China National Standards, GB/T 1454-2021. (in Chinese) [13] 王凯, 贺强. 聚甲基丙烯酰亚胺泡沫夹层结构全生命周期的关键技术研究进展[J]. 复合材料学报, 2020, 37(8): 1805-1822.WANG K, HE Q. Progress on study of key technologies for polymethacrylimide foam core sandwich lifecycle[J]. Acta Materiae Compositae Sinica, 2020, 37(8): 1805-1822(in Chinese). [14] 戴福洪, 王广宁. 埋微带天线蜂窝夹层结构的力电性能分析[J]. 复合材料学报, 2011, 28(02): 231-234.DAI H, WANG G, Analysis of mechanical and electric performance of honeycomb sandwich structures embedded with the microstrip antenna[J]. Acta Materiae Compositae Sinica, 2011, 28(02): 231-234. (in Chinese) [15] SONG S, XIONG C, ZHENG J, et al. Compression, bending, energy absorption properties, and failure modes of composite kagome honeycomb sandwich structure reinforced by PMI foams[J]. Composite Structures, 2021, 277. [16] 陈祥宝. 聚合物基复合材料手册[M]. 聚合物基复合材料手册, 2004, 328-332.CHEN X. Handbook of Polymer Matrix Composite Materials[M]. Handbook of Polymer Matrix Composite Materials, 2004. (in Chinese) [17] 段苗苗, 贾坤荣, 宋千光. 蜂窝夹层共形承载天线结构剪切性能的试验与模拟[J]. 工程塑料应用, 2017, 45(7): 61-64+72. doi: 10.3969/j.issn.1001-3539.2017.07.012DUAN M, JIA K, SONG Q. Test and simulation of shear performance of honeycomb sandwich conformal load-bearing antenna structure[J]. Engineering Plastics Application, 2017, 45(7): 61-64+72(in Chinese). doi: 10.3969/j.issn.1001-3539.2017.07.012 [18] ESNAOLA A, ELGUEZABAL B, AURREKOETXEA J, et al. Optimization of the semi-hexagonal geometry of a composite crush structure by finite element analysis[J]. Composites Part B: Engineering, 2016, 93: 56-66. doi: 10.1016/j.compositesb.2016.03.002 [19] CHEN Y, YE L, FU K, et al. Transition from buckling to progressive failure during quasi-static in-plane crushing of CF/EP composite sandwich panels[J]. Composites Science and Technology, 2018, 168: 133-144. doi: 10.1016/j.compscitech.2018.09.017 [20] HASHIN, Z. Failure criteria for unidirectional fiber composites[J]. Journal of Applied Mechanics-Transactions of the ASME, 1980, 47(2): 329-334. doi: 10.1115/1.3153664 [21] TAY T E, LIU G, TAN V B C, et al. Progressive failure analysis of composites[J]. Journal of Composite Materials, 2008, 42(18): 1921-1966. doi: 10.1177/0021998308093912 [22] MAIMI P, CAMANHO P R, MAYUGO J A, et al. A continuum damage model for composite laminates: Part II - Computational implementation and validation[J]. Mechanics of Materials, 2007, 39(10): 909-919. doi: 10.1016/j.mechmat.2007.03.006 [23] MI Y, CRISFIELD M A, DAVIES G A O, et al. Progressive delamination using interface elements[J]. Journal of Composite Materials, 1998, 32(14): 1246-1272. doi: 10.1177/002199839803201401 [24] TSAO C C. Experimental study of drilling composite materials with step-core drill[J]. Materials & Design, 2008, 29(9): 1740-1744. [25] 郑权, 韩涵, 唐杰等. 含胶接缺陷的椭球泡沫夹层结构破坏机理与承载能力研究[J]. 实验力学, 2015, 30(2): 207-214. doi: 10.7520/1001-4888-14-081ZHENG Q, HAN H, TANG J, et al. On the failure mechanism and bearing capacity of ellipsoidal foam core sandwich structure with adhesive bonding defects[J]. Journal of Experimental Mechanics, 2015, 30(2): 207-214(in Chinese). doi: 10.7520/1001-4888-14-081 [26] NAGLER M, THOR M, PEYRER P, et al. Analysis of the macroscopic behaviour of PMI foam[J]. Key Engineering Materials, 2019, 809: 285-290. doi: 10.4028/www.scientific.net/KEM.809.285 [27] 王杰. 复合材料泡沫夹层结构低速冲击与冲击后压缩性能研究[D]. 上海交通大学, 2013.WANG J. Study on the low-velocity imapct and compression-after-impact behavior of foam-core sandwich panels[D]. Shanghai Jiao Tong University, 2013. (in Chinese) -
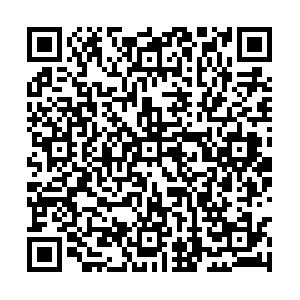
计量
- 文章访问数: 36
- HTML全文浏览量: 7
- 被引次数: 0