Accelerated aging behavior of glass fiber reinforced methacrylate-based cured-in-place-pipe lining in seawater and sulfuric acid
-
摘要: 原位固化管道(Cured-in-place-pipe, CIPP)内衬用于修复被生物硫酸腐蚀的排水管道,也用于市政排海管道。而玻璃纤维/甲基丙烯酸酯基复合材料用于CIPP内衬,在硫酸和海水环境下的耐久性尚不明确。设计了0.5%硫酸、模拟海水与高温(80℃)加速相耦合的老化实验,以纯水作为对照,基于吸水测试、三点弯曲测试、接触角分析、SEM和FTIR等表征测试方法,研究了玻璃纤维/甲基丙烯酸酯基CIPP内衬的老化行为。结果显示:0.5%硫酸、模拟海水和纯水加速老化1440 h后,弯曲强度分别下降了57.9%、58.4%和57.4%,而弯曲模量没有明显下降;酯键水解生成的羟基部分被氧化为羰基,使树脂老化后颜色发黄;硫酸劣化了树脂表面使润湿性降低,也通过腐蚀表面玻璃纤维促进了水分扩散;海水中盐分析出结晶阻碍了水分扩散,也严重破坏了材料表面的树脂层,也使润湿性增强。提高CIPP内衬的耐久性,重点应该抑制水分扩散劣化界面。可为甲基丙烯酸酯用于CIPP修复材料的耐久性评估提供参考依据。Abstract: Cured-in-place-pipe (CIPP) lining is used to repair drainage pipes corroded by biological sulfuric acid and is also used in municipal sea drainage pipes. However, the durability of glass fiber reinforced methacrylate-based composite materials used in CIPP lining in sulfuric acid and seawater environments is unclear. This study established two aging conditions: 0.5% sulfuric acid and simulated seawater, with the temperature of 80°C to accelerate aging. Pure water was used as a control. Characterization and testing methods such as water absorption test, three-point bending test, contact angle analysis, SEM, and FTIR were used for the study to evaluate the aging behavior of glass fiber reinforced methacrylate-based CIPP lining. The results show that the bending strength decreases by 57.9%, 58.4%, and 57.4% respectively, after accelerated aging in 0.5% sulfuric acid, simulated seawater, and pure water for 1440 hours. While the bending modulus does not show a significant decrease. The hydroxyl group generated by the hydrolysis of the ester bond is partially oxidized to a carbonyl group, causing the resin to turn yellow after aging. Sulfuric acid deteriorates the resin surface and reduces the wettability, and also promotes water diffusion by corroding the surface glass fiber. The crystallization of salt in seawater hinders the diffusion of water and severely damages the resin layer on the material surface, leading to enhanced wettability. To enhance CIPP lining durability, efforts should focus on inhibiting moisture diffusion and degradation at the interface. This study can provide a reference basis for the durability evaluation of methacrylate used in CIPP repair materials.
-
表 1 模拟海水成分
Table 1. Simulated seawater composition
Compounds Concentration /(g·L−1) NaCl 24.53 MgCl2 5.20 Na2SO4 4.09 CaCl2 1.16 KCl 0.695 NaHCO3 0.201 KBr 0.101 H3BO3 0.027 SrCl2 0.025 NaF 0.003 表 2 玻璃纤维/甲基丙烯酸酯基CIPP内衬加速老化1440 h前后的羰基指数和羟基指数
Table 2. The carbonyl index and hydroxyl index of glass fiber reinforced methacrylate-based CIPP lining before and after accelerated aging1440 h
Carbonyl index Hydroxyl index Before aging Aging 1440 h Before aging Aging 1440 h 0.5% Sulfuric acid 0.560±0.025 0.807±0.026 0±0.01 0.038±0.028 Simulated seawater 0.591±0.019 0.056±0.023 Pure water 0.824±0.021 0.039±0.035 -
[1] DONALDSON B M. Environmental implications of cured-in-place pipe rehabilitation technology[J]. Transportation research record, 2009, 2123(1): 172-179. doi: 10.3141/2123-19 [2] ZHU H, WANG T, WANG Y, et al. Trenchless rehabilitation for concrete pipelines of water infrastructure: A review from the structural perspective[J]. Cement and Concrete Composites, 2021, 123: 104193. doi: 10.1016/j.cemconcomp.2021.104193 [3] LI S, GUO S C, YAO Y M, et al. The effects of aging in seawater and SWSSC and strain rate on the tensile performance of GFRP/BFRP composites: A critical review[J]. Constr Build Mater, 2021, 282. [4] NURUDDIN M, DECOCKER K, SENDESI S M T, et al. Influence of aggressive environmental aging on mechanical and thermo-mechanical properties of Ultra Violet (UV) Cured in Place Pipe liners[J]. Journal of Composite Materials, 2020, 54(23): 3365-3379. doi: 10.1177/0021998320913988 [5] RA K, SENDESI S M T, NURUDDIN M, et al. Considerations for emission monitoring and liner analysis of thermally manufactured sewer cured-in-place-pipes (CIPP)[J]. Journal of hazardous materials, 2019, 371: 540-549. doi: 10.1016/j.jhazmat.2019.02.097 [6] GRAS-TRAVESSET F, ANDREU-TORRAS A, PéREZ M A. A novel test procedure for evaluating the performance of composite cured-in-place-pipe liners in water pressure pipe rehabilitation[J]. Case Studies in Construction Materials, 2023, 19: e02381. doi: 10.1016/j.cscm.2023.e02381 [7] VAHIDI E, JIN E, DAS M, et al. Environmental life cycle analysis of pipe materials for sewer systems[J]. Sustainable Cities and Society, 2016, 27: 167-174. doi: 10.1016/j.scs.2016.06.028 [8] ALLOUCHE E, ALAM S, SIMICEVIC J, et al. A pilot study for retrospective evaluation of cured-in-place pipe (CIPP) rehabilitation of municipal gravity sewers[J]. Tunnelling and Underground Space Technology, 2014, 39: 82-93. doi: 10.1016/j.tust.2012.02.002 [9] OKABE S, ODAGIRI M, ITO T, et al. Succession of sulfur-oxidizing bacteria in the microbial community on corroding concrete in sewer systems[J]. Applied and environmental microbiology, 2007, 73(3): 971-980. doi: 10.1128/AEM.02054-06 [10] VALIX M, SHANMUGARAJAH K. Biogenic acids produced on epoxy linings installed in sewer crown and tidal zones[J]. Water research, 2015, 80: 217-226. doi: 10.1016/j.watres.2015.05.027 [11] 魏建辉, 刘明, 高进城, 等. 吸湿老化后碳纤维增强乙烯基脂树脂复合材料高低温力学性能[J]. 复合材料学报, 2023, 40(6): 3279-3290.WEI Jianhui, LIU Ming, GAO Jincheng, et al. Mechanical properties at elevated and cryogenic temperatures of carbon fiber reinforced vinylester resin composites after hygroscopic aging[J]. Acta Materiae Compositae Sinica, 2023, 40(6): 3279-3290(in Chinese). [12] NODEHI M. Epoxy, polyester and vinyl ester based polymer concrete: a review[J]. Innovative Infrastructure Solutions, 2021, 7(1): 64. [13] VIEIRA P S, DA SILVA G A, LOPES B J, et al. Hygrothermal aging of steel/frp pipe repair systems: A literature review[J]. International Journal of Pressure Vessels and Piping, 2023, 201: 104881. doi: 10.1016/j.ijpvp.2022.104881 [14] 高坤, 史汉桥, 孙宝岗, 等. 湿热老化对玻璃纤维/环氧树脂复合材料性能的影响[J]. 复合材料学报, 2016, 33(6): 1147-1152.GAO Kun, SHI Hanqiao, SUN Baogang, et al. Effects of hydro-thermal aging on properties of glass fiber/epoxy composites[J]. Acta Materiae Compositae Sinica, 2016, 33(6): 1147-1152(in Chinese). [15] AITHAL S, HOSSAGADDE P N, KINI M V, et al. Durability study of quasi-isotropic carbon/epoxy composites under various environmental conditions[J]. Iranian Polymer Journal, 2023, 32(7): 873-885. doi: 10.1007/s13726-023-01172-x [16] VISCO A M, BRANCATO V, CAMPO N. Degradation effects in polyester and vinyl ester resins induced by accelerated aging in seawater[J]. Journal of Composite Materials, 2011, 46(17): 2025-2040. [17] HE W, LI X, LI P, et al. Experimental Investigation on Hygroscopic Aging of Glass Fiber Reinforced Vinylester Resin Composites[J]. Polymers, 2022, 14(18): 3828. doi: 10.3390/polym14183828 [18] 曹银龙, 于桢琪, 冯鹏, 等. 纤维增强环氧/乙烯基树脂复合材料性能优化与劣化机制研究进展[J]. 复合材料学报, 2024, 41(3): 1179-1191.CAO Yinlong, YU Zhenqi, FENG Peng, et al. Performance optimization and deterioration mechanism of fiber reinforced epoxy/vinyl resin composite materials: A review[J]. Acta Materiae Compositae Sinica, 2024, 41(3): 1181-1193(in Chinese). [19] JOSé-TRUJILLO E, RUBIO-GONZáLEZ C, RODRíGUEZ-GONZáLEZ J. Seawater ageing effect on the mechanical properties of composites with different fiber and matrix types[J]. Journal of Composite Materials, 2019, 53(23): 3229-41. doi: 10.1177/0021998318811514 [20] KANERVA M, JOKINEN J, SARLIN E, et al. Lower stiffness of GFRP after sulfuric acid-solution aging is due to degradation of fibre-matrix interfaces?[J]. Composite Structures, 2019, 212: 524-534. doi: 10.1016/j.compstruct.2019.01.006 [21] LI H, GU P, WATSON J, et al. Acid corrosion resistance and mechanism of E-glass fibers: boron factor[J]. Journal of Materials Science, 2013, 48(8): 3075-3087. doi: 10.1007/s10853-012-7082-y [22] 韦嘉盛, 戴磊, 贺瓶. 基于纤维素及其衍生物的凝胶材料设计[J]. 复合材料学报, 2022, 39(7): 3084-3103.WEI Jiasheng, DAI Lei, HE Ping. Design of gel materials with cellulose and its derivatives[J]. Acta Materiae Compositae Sinica, 2022, 39(7): 3084-3103(in Chinese). [23] DEVINE M, BAJPAI A, OBANDE W, et al. Seawater ageing of thermoplastic acrylic hybrid matrix composites for marine applications[J]. Composites Part B: Engineering, 2023, 263: 110879. doi: 10.1016/j.compositesb.2023.110879 [24] DAVIES P, LE GAC P-Y, LE GALL M. Influence of sea water aging on the mechanical behaviour of acrylic matrix composites[J]. Applied Composite Materials, 2017, 24(1): 97-111. doi: 10.1007/s10443-016-9516-1 [25] DUO Y, LIU X, LIU Y, et al. Environmental impact on the durability of FRP reinforcing bars[J]. Journal of Building Engineering, 2021, 43: 102909. doi: 10.1016/j.jobe.2021.102909 [26] VISCO A, BRANCATO V, CAMPO N. Degradation effects in polyester and vinyl ester resins induced by accelerated aging in seawater[J]. Journal of Composite Materials, 2012, 46(17): 2025-2040. doi: 10.1177/0021998311428533 [27] American Society for Testing and Materials International. Standard Practice for Preparation of Substitute Ocean Water: ASTM D1141-98(2021) [S]. West Conshohocken: ASTM International, 2021. [28] HONG B, XIAN G, LI H. Comparative study of the durability behaviors of epoxy-and polyurethane-based CFRP plates subjected to the combined effects of sustained bending and water/seawater immersion[J]. Polymers, 2017, 9(11): 603. doi: 10.3390/polym9110603 [29] GRAMMATIKOS S A, EVERNDEN M, MITCHELS J, et al. On the response to hygrothermal aging of pultruded FRPs used in the civil engineering sector[J]. Materials & Design, 2016, 96: 283-295. [30] 吴瑞, 李岩, 于涛. 不同种类纤维增强复合材料湿热老化性能对比[J]. 复合材料学报, 2022, 39(9): 4406-4419.WU Rui, LI Yan, YU Tao. Comparative study on the hygrothermal durability of different fiber reinforced composites[J]. Acta Materiae Compositae Sinica, 2022, 39(9): 4406-4419(in Chinese). [31] American Society for Testing and Materials International. Standard Test Method for Flexural Properties of Polymer Matrix Composite Materials: ASTM D7264/D7264M-21 [S]. West Conshohocken: ASTM International, 2021. [32] AWAD S A, FELLOWS C M, SAEED MAHINI S. A comparative study of accelerated weathering of epoxy resins based on DGEBA and HDGEBA[J]. Journal of Polymer Research, 2018, 25: 1-8. doi: 10.1007/s10965-017-1408-z [33] ASLAN A, SALUR E, DüZCüKOĞLU H, et al. The effects of harsh aging environments on the properties of neat and MWCNT reinforced epoxy resins[J]. Constr Build Mater, 2021, 272: 121929. doi: 10.1016/j.conbuildmat.2020.121929 [34] FANG Y, WANG K, HUI D, et al. Monitoring of seawater immersion degradation in glass fibre reinforced polymer composites using quantum dots[J]. COMPOSITES PART B-ENGINEERING, 2017, 112: 93-102. doi: 10.1016/j.compositesb.2016.12.043 [35] LIU T, LIU X, FENG P. A comprehensive review on mechanical properties of pultruded FRP composites subjected to long-term environmental effects[J]. Composites Part B: Engineering, 2020, 191: 107958. doi: 10.1016/j.compositesb.2020.107958 [36] BEURA S, CHAKRAVERTY A, PATI S, et al. Effect of salinity and strain rate on sea water aged GFRP composite for marine applications[J]. Materials Today Communications, 2023, 34: 105056. doi: 10.1016/j.mtcomm.2022.105056 [37] FENG Z, SONG G-L, WANG Z M, et al. Salt crystallization-assisted degradation of epoxy resin surface in simulated marine environments[J]. Progress in Organic Coatings, 2020, 149: 105932. doi: 10.1016/j.porgcoat.2020.105932 [38] MA Y, YANG Y, SUGAHARA T, et al. A study on the failure behavior and mechanical properties of unidirectional fiber reinforced thermosetting and thermoplastic composites[J]. Composites Part B: Engineering, 2016, 99: 162-172. doi: 10.1016/j.compositesb.2016.06.005 [39] WU C, MENG B C, TAM L-H, et al. Yellowing mechanisms of epoxy and vinyl ester resins under thermal, UV and natural aging conditions and protection methods[J]. Polymer Testing, 2022, 114: 107708. doi: 10.1016/j.polymertesting.2022.107708 [40] SMITH B. Infrared Spectroscopy of Polymers, VIII: Polyesters and the Rule of Three[J]. Spectroscopy, 2022, 37(10): 25-28. [41] ARRIETA J S, RICHAUD E, FAYOLLE B, et al. Thermal oxidation of vinyl ester and unsaturated polyester resins[J]. Polymer Degradation and Stability, 2016, 129: 142-155. doi: 10.1016/j.polymdegradstab.2016.04.003 [42] BAKLAN D, BILOUSOVA A, MYRONYUK O. UV aging of styrene-acrylic polymer SiO2 and TiO2 composites[J]. Materials Today Communications, 2024, 38: 107990. doi: 10.1016/j.mtcomm.2023.107990 [43] YUAN Y, HAYS M P, HARDWIDGE P R, et al. Surface characteristics influencing bacterial adhesion to polymeric substrates[J]. RSC advances, 2017, 7(23): 14254-14261. doi: 10.1039/C7RA01571B [44] WANG J, WU Y, CAO Y, et al. Influence of surface roughness on contact angle hysteresis and spreading work[J]. Colloid and Polymer Science, 2020, 298(8): 1107-1112. doi: 10.1007/s00396-020-04680-x [45] SANCHIS M, BLANES V, BLANES M, et al. Surface modification of low density polyethylene (LDPE) film by low pressure O2 plasma treatment[J]. European Polymer Journal, 2006, 42(7): 1558-1568. doi: 10.1016/j.eurpolymj.2006.02.001 -
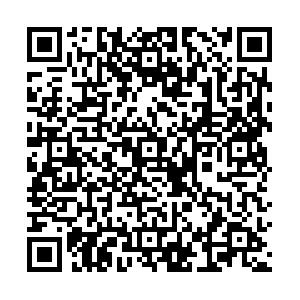
计量
- 文章访问数: 114
- HTML全文浏览量: 57
- 被引次数: 0