Full-field fiber trajectory motion simulation and distribution verification of complex components based on three-dimensional winding technology
-
摘要: 三维缠绕技术是一种新兴的复合材料预成型体制造技术,可以通过机器人辅助缠绕解决复杂芯模大角度纤维铺放难题,在一定长度内对任意几何形状芯模做环形缠绕。但是三维缠绕的控制方式还不成熟,对异型芯模的缠绕轨迹较为复杂,所得的纤维轨迹也难以预测。经过对三维缠绕纤维沉积过程的研究,采用运动学仿真法建立三维缠绕仿真模型,实现对三维缠绕纤维轨迹的快速预测。首先对异型芯模进行网格重构,提升了仿真的精度;然后利用空间离散点位模拟围绕复杂芯模时丝嘴的运动轨迹;最后利用纤维沉积机制作为判据,实现仿真纤维轨迹的迭代更新。三维缠绕仿真模型有两个主要输入参数:旋转环步进距离与旋转环偏转角度。通过现场试验验证,步进距离增大时,芯模的面密度会稳定降低,旋转环的动态偏转也会直接影响到弯曲芯模内外侧纤维轨迹。对直芯模进行运动学仿真所得试验结果与纤维沉积理论计算值对比,误差小于0.2%。理论与实践相结合的研究证明了运动学仿真法对三维缠绕技术仿真的可靠性与准确性。Abstract: As an emerging composite preform manufacturing technology, three-dimensional winding technology can solve the problem of large-angle fiber placement for complex core molds and make circular winding for core molds with arbitrary shapes within a certain length by robot-assisted winding. However, the control method of 3D winding is still immature, the winding trajectory of the shaped core mold is complicated, and the resulting fiber structure is difficult to predict. After the study of 3D winding fiber deposition process, the kinematic simulation method was used to establish a 3D winding simulation model to realize the fast prediction of 3D winding fiber structure. Firstly, the mesh reconstruction of the shaped mandrel was carried out to improve the accuracy of the simulation; then the spatial discrete point positions were used to simulate the motion trajectory of the filament nozzle around the complex mandrel, and finally the fiber deposition mechanism was used as a criterion to achieve the iterative updating of the simulated fiber structure. The 3D winding simulation model has two main control parameters: the step and the deflection angle of the rotary head. It is verified through experiments that the surface density of the mandrel decreases when the step increases, and the dynamic deflection of the rotary head directly affects the inner and outer fiber structure of the curved mandrel. The experiment results obtained from the simulation of the straight mandrel are compared with the theoretically calculated values, and the error is less than 0.2%. The reliability and accuracy of the kinematic simulation method for the simulation of 3D winding technology is proved by the combination of theoretical and practical research.
-
图 4 (a)对不规则网格模型等距切片;(b)模型切片后得到的表面轮廓点位;(c)利用点位重构网格;(d)对其他形状芯模进行网格重构
Figure 4. (a) Equally spaced slicing of an irregular mesh model; (b) Surface contour point locations obtained after slicing the model; (c) Reconstruction of the mesh using the point locations; (d) Mesh reconstruction for other shapes of core molds
图 10 (a)矩形直管直螺旋缠绕仿真;(b)矩形直管斜螺旋缠绕仿真;(c)矩形弯管螺旋缠绕仿真;(d)矩形直管直螺旋缠绕沉积点与几何尺寸;(e)矩形直管斜螺旋缠绕沉积点与几何尺寸;(f)在矩形弯管上仿真的沉积纤维
Figure 10. (a) Rectangular straight tube positive helical winding simulation; (b) Rectangular straight tube inclined helical winding simulation; (c) Rectangular bent tube helical winding simulation; (d) Rectangular straight tube positive helical winding deposition point and geometry; (e) Rectangular straight tube inclined helical winding deposition point and geometry; (f) Deposited fibers simulated on a rectangular bent tube
表 1 矩形四面的缠绕角仿真与数值计算对比
Table 1. Comparison between simulation and numerical calculation of winding angle for rectangular four faces
Face numbering Straight spiral track winding Inclined spiral track winding Theoretical/(°) Simulation/(°) Error/% Theoretical/(°) Simulation/(°) Error/% 1 88.1757 88.1763 6.805×10−4 98.1945 98.2026 8.249×10−3 2 88.2578 88.2608 3.399×10−3 88.2141 88.2579 4.965×10−2 3 88.1757 88.1763 6.805×10−4 78.2557 78.2361 −2.505×10−2 4 88.2578 88.2608 3.399×10−3 88.2141 88.3281 0.1292 -
[1] Rajak DK, Wagh PH, Linul E. Manufacturing Technologies of Carbon/Glass Fiber-Reinforced Polymer Composites and Their Properties: A Review[J]. 2021. Polymers, 2021, 13(21): 3721. [2] 郝大贤, 王伟, 王琦珑, 等. 复合材料加工领域机器人的应用与发展趋势[J]. 机械工程学报, 2019, 55(3): 1-17. doi: 10.3901/JME.2019.03.001HAO Daxian, WANG Wei, WANG Qilong, et al. Applications and Development Trend of Robotics in Composite Material Process[J]. Journal of Mechanical Engineering, 2019, 55(3): 1-17(in Chinese). doi: 10.3901/JME.2019.03.001 [3] Azeem M, Ya HH, Alam MA, et al. Application of Filament Winding Technology in Composite Pressure Vessels and Challenges: A Review[J]. Journal of Energy Storage, 2022, 49: 103468. doi: 10.1016/j.est.2021.103468 [4] Özbek Ö, Kiliç A, Bozkurt ÖY. Development of Filament Winding Machine for Producing Round Shapes with Different Fiber Reinforcements[J]. Gümüşhane Üniversitesi Fen Bilimleri Enstitüsü Dergisi, 2020, 10(3): 552-558. [5] 周强. 编织-缠绕-拉挤一体化生产线缠绕系统设计与分析[D]. 东华大学, 2024.ZHOU Qiang. Design and analysis of winding system for integrated knitting-winding-pultrusion production line[D]. Donghua University, 2024(in Chinese). [6] 缑依锟, 段跃新, 宁博, 等. 捆绑纱线密度变化对经编织物复合材料性能的影响[J]. 复合材料科学与工程, 2023, (4): 21–26, 33.GOU Yikun, DUAN Yuexin , NING Bo, et al. Effect of binding yarn linear density change on properties of non-crimp fabrics composites[J]. Composites Science And Engineering, 2023, (4): 21–26, 33(in Chinese). [7] 祖磊, 穆建桥, 王继辉, 等. 基于非测地线纤维缠绕压力容器线型设计与优化[J]. 复合材料学报, 2016, 33(5): 1125-1131.ZU L, MU J Q, WANG J H, et al. Pattern design and optimization of filament winding pressure vessels based on non-geodesics[J]. Acta Materiae Compositae Sinica, 2016, 33(5): 1125-1131 (in Chinese). [8] HARPER L, CLIFFORD M. Design and manufacturing of structural composites[M]. 2023: 170-175. [9] 阎冬. 基于工业机器人的预浸带缠绕装备设计与研究[D]. 武汉理工大学, 2021.YAN Dong, Design and Research of Prepreg Tape Winding Equipment Based on Industrial Robot[D]. Wuhan University of Technology, 2021(in Chinese). [10] 杨海. 复合材料纤维缠绕机器人关键技术研究[D]. 哈尔滨理工大学, 2020.YANG Hai, Research on Key Technologies of Filament Winding Robot of Composite[D]. Harbin University of Science and Technology, 2020(in Chinese). [11] 马咏昊. 复合材料连接件的计算机辅助缠绕线型设计方法[D]. 华东师范大学, 2024.MA YongHao, Computer Aided Filament Winding For Composite[D]. East China Normal University, 2024(in Chinese). [12] Kessels JFA, Akkerman R. Prediction of the yarn trajectories on complex braided preforms[J]. Composites Part A: Applied Science and Manufacturing, 2002, 33(8): 1073-1081. doi: 10.1016/S1359-835X(02)00075-1 [13] Akkerman R, Rodriguez B. Braiding simulation for RTM preforms[J]. IEEE Transactions on Magnetics - IEEE TRANS MAGN, 2006. [14] Van Ravenhorst JH, Akkerman R. Circular braiding take-up speed generation using inverse kinematics[J]. Composites Part A: Applied Science and Manufacturing, 2014, 64: 147-158. doi: 10.1016/j.compositesa.2014.04.020 [15] Gondran M, Abdin Y, Gendreau Y, et al. Automated braiding of non-axisymmetric structures using an iterative inverse solution with angle control[J]. Composites Part A: Applied Science and Manufacturing, 2021, 143: 106288. doi: 10.1016/j.compositesa.2021.106288 [16] Monnot P, Lévesque J, Laberge Lebel L. Automated braiding of a complex aircraft fuselage frame using a non-circular braiding model[J]. Composites Part A: Applied Science and Manufacturing, 2017, 102: 48-63. doi: 10.1016/j.compositesa.2017.07.011 [17] Vu AN, Grouve WJB, Akkerman R. Modeling of yarn interactions for non-axisymmetric biaxial overbraiding simulations[J]. Composites Part A: Applied Science and Manufacturing, 2023, 167: 107421. doi: 10.1016/j.compositesa.2022.107421 [18] Li C, Li Q, Chi X, et al. Design of fast braiding process based on reconstructing mesh with complex and irregular mandrels[J]. Textile Research Journal, 2024: 00405175241232142. [19] Li Q, Chi X, Ji C, et al. Off-center braiding process for complex composite preforms based on analysis of the geometric contour model of the mandrel[J]. Textile Research Journal, 2022, 92(23-24): 4845-4859. doi: 10.1177/00405175221108523 [20] 李麒阳, 季诚昌, 郗欣甫, 等. 大尺寸异形结构芯模编织策略及纱线轨迹预测[J]. 纺织学报, 2023, 44(10): 188-195.LI Qiyang, JI Chengchang, CHI Xinfu, et al. Braiding strategy and yarn trajectory prediction of large size special-shaped structure mandrel[J]. Journal of Textile Research, 2023, 44(10): 188-195(in Chinese). [21] 张行, 任明法, 王磊, 等. 纤维缠绕复合材料压力容器封头厚度的逐层预测方法[J]. 复合材料学报, 2024, 41(7): 3797-3803.ZHANG H, REN M F, WANG L, et al. A method for predicting dome thickness layer by layer of filament wound composite pressure vessel[J]. Acta Materiae Compositae Sinica, 2024, 41(7): 3797-3803(in Chinese). [22] 郭凯特, 王春, 文立华, 等. 不等开口纤维增强树脂复合材料缠绕壳体非测地线线型设计[J]. 复合材料学报, 2019, 36(5): 1189-1199.Guo Kaite, WANG Chun, WEN Lihua, et al. Winding pattern design of fiber reinforced resin polymer composites winding vessels with unequal polar openings based on non-geodesics[J]. Acta Materiae Compositae Sinica, 2019, 36(5): 1189-1199 (in Chinese). [23] Tang J, Wang J, Zhao G, et al. 3D winding path modeling on truncated conical shell with proposed outer-contour expanding and convex helix algorithms[J]. Composite Structures, 2023, 305: 116484. doi: 10.1016/j.compstruct.2022.116484 [24] KYOSEV Y. Advances in braiding technology: specialized techniques and applications[M]. 2016: 464. [25] Ravenhorst JHV. Design tools for circular overbraiding of complex mandrels[D]. 2018. [26] 张智云. 二次螺旋斜弹簧的数学建模[J]. 机电元件, 2018, 38(5): 25-28.ZHANG ZhiYun. Mathematical modeling of secondary helical inclined springs[J]. Electromechanical Components, 2018, 38(5): 25-28(in Chinese). -
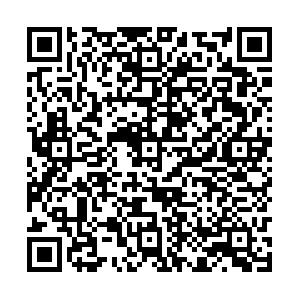
计量
- 文章访问数: 29
- HTML全文浏览量: 23
- 被引次数: 0