Ru sensitized MOF electronic structure for efficient water electrolysis
-
摘要: 金属有机框架(MOF)材料因其强大的结构和功能可调性已成为极具潜力的电解水催化剂。然而,MOF催化剂由于自身较弱的电荷转移能力及有限的稳定性,其固有活性较低。因此,设计出兼具高活性与高稳定性的MOF催化剂仍是具有挑战性的难题。本文通过两步水热法成功合成了CoRu-BDC/NF(BDC:对苯二甲酸、NF:泡沫镍),Ru的引入调节了负载在NF上Co-BDC的内在活性,赋予了CoRu-BDC/NF丰富的活性位点、较快的电荷传输能力,有利于电催化性能的提升。结果表明,CoRu-BDC/NF在酸性环境中电流密度达到10 mA·cm−2时,HER过电位为34 mV,Tafel斜率为33 mV·dec−1。值得注意的是,在碱性条件下,当电流密度为10 mA·cm−2,HER和OER所需的过电位也仅为32 mV和280 mV,Tafel斜率分别为39 mV·dec−1 、49 mV·dec−1,均表现出优异的电催化性能。Ru的引入导致Co的电子结构和配位环境发生变化,使原始Co-MOF的表面积增加,为电化学反应提供了更好的电子转移平台,加快了电子在电极和电解液界面间的传递,进一步提升了电催化性能。Abstract: Metal-organic framework (MOF) materials have become potential catalysts for water electrolysis due to their strong structural and functional adjustability. However, the intrinsic activity of MOF catalyst is low due to its weak charge transfer ability and limited stability. Therefore, it is still a challenging problem to design MOF catalysts with high activity and high stability. In this paper, CoRu-BDC/NF(BDC: terylene acid, NF: nickel foam) was successfully synthesized by two-step hydrothermal method. The introduction of Ru regulated the intrinsic activity of Co-BDC loaded on NF, endowed CoRu-BDC/NF with abundant active sites and fast charge transport capacity, which was conducive to the improvement of electrocatalytic performance. The results show that when the current density of CoRu-BDC/NF reaches 10 mA·cm−2 in acidic environment, HER overpotential is 34 mV and Tafel slope is 33 mV·dec−1. It is worth noting that under alkaline conditions, when the current density is 10 mA·cm−2, the required overpotential of HER and OER is only 32 mV and 280 mV, and the slope of Tafel is 39 mV·dec−1 and 49 mV·dec−1, showing excellent electrocatalytic performance. The introduction of Ru leads to changes in the electronic structure and coordination environment of Co, increasing the surface area of the original Co-MOF, providing a better electron transfer platform for the electrochemical reaction, speeding up the transfer of electrons between the electrode and the electrolyte interface, and further improving the electrocatalytic performance.
-
Key words:
- CoRu-BDC /
- Ru introduction /
- HER /
- OER /
- coordinated environment
-
图 1 (a-d)分别CoRu-BDC/NF纳米复合材料的FE-SEM图像;(e-h,k)为CoRu-BDC的TEM图;(i-j)为CoRu-BDC的TEM-EDX图;(l)为CoRu-BDC电子衍射图
Figure 1. Fig 1(a-d) are the FE-SEM images of CoRu-BDC/NF nanocomposites, respectively. (e-h,k-i) indicates the TEM image of CoRu-BDC, (i-j) is the TEM-EDX image of CoRu-BDC
BDC—Terylene acid; NF—NAickel foam
图 2 Co-BDC和CoRu-BDC(a)XRD图;(b)拉曼图;(c)傅里叶变换红外光谱;(d) XPS全谱图;(e) Co 2p轨道的高分辨XPS图;(f) Ru 3d轨道的高分辨XPS图
Figure 2. Co-BDC and CoRu-BDC (a) XRD; (b) Raman map; (c) Fourier transform infrared spectrum; (d) XPS full spectrum; (e) high resolution XPS map of Co 2p orbit; (f) high resolution XPS map of Ru 3d orbit
图 3 NF、Co-BDC/NF、Ru-BDC/NF、CoRu-BDC/NF在0.5 mol/L H2SO4中的电化学HER测量:(a) 极化曲线;(b) Tafel斜率; (c) CoRU-BDC/NF不同扫速下的CV曲线; (d) 0.25 V (vs.RHE)处电流密度与扫速的差值; (e) 阻抗图;(f)HER过程中CoRu-BDC/NF的稳定性
Figure 3. Electrochemical HER measurements of NF, Co-BDC/NF, Ru-BDC/NF and CoRu-BDC/NF in 0.5 M H2SO4 . (a) Polarization curves, (b) Tafel plots,(c) CV curves at different sweeps of CoRu-BDC/NF speed, (d) Difference of current density at 0.25 V( vs.RHE) as a function of scan rate, (e) Nyquist plots, (f) Stability of the CoRu-BDC/NF during the HER process
j—Current density; E —Overpotentials; Z’ —Impedance the real part; Z’’ —Impedance virtual part
图 5 NF、Co-BDC/NF、Ru-BDC/NF和CoRu-BDC/NF:(a)在1 mol/L KOH中的电化学OER测试的极化曲线;(b) Tafel斜率; (c)阻抗图;(d) CoRU-BDC/NF不同扫速下的CV曲线; (e) 0.25 V (vs.RHE)处电流密度与扫速的差值;(f) CoRu-BDC/NF||CoRu-BDC/NF以5 mV·s−1在1 mol/L KOH整体水分的极化曲线。
Figure 5. NF, Co-BDC/NF, Ru-BDC/NF and CoRu-BDC/NF. (a) Polarization curves for the electrochemical OER tests in 1 M KOH, (b)Tafel plots, (c) Nyquist plots, (d) CV curves at different sweeps of CoRu-BDC/NF speed, (e) Difference of current density at 0.25 V (vs.RHE) as a function of scan rate, (e) Polarization curves for CoRu-BDC/NF||CoRu-BDC/NF couples toward overall water splitting recorded at a scan rate of 5 mV s −1 in 1 M KOH.
表 1 近期报道的相关材料全解水性能的比较
Table 1. Comparison of the recent reported overall water solution properties of related materials
Electrocatalyst Acid conditions
HER η10/mVAlkaline conditions
HER η10/mVAlkaline conditions
OER η10/mVOverall Water/V Reference CoRu-BDC/NF 34 32 280 1.47 This work Co-Co2C/CC / 96 261 1.63 [51] CoFeP-N / 64 219 1.56 [52] CoCu@NC / 199 301 1.87 [53] Co-CN@NiFe / 87 233 1.55 [54] Notes:η10—Overpotentials with a current density of 10 mA·cm−2 -
[1] MENG C, CAO Y, LUO Y, et al. A Ni-MOF nanosheet array for efficient oxygen evolution electrocatalysis in alkaline media[J]. Inorganic Chemistry Frontiers, 2021, 8(12): 3007-3011. doi: 10.1039/D1QI00345C [2] JIAO L, ZHU J, ZHANG Y, et al. Non-Bonding Interaction of Neighboring Fe and Ni Single-Atom Pairs on MOF-Derived N-Doped Carbon for Enhanced CO(2) Electroreduction[J]. J Am Chem Soc, 2021, 143(46): 19417-19424. doi: 10.1021/jacs.1c08050 [3] CHEN C, SUN M, ZHANG F, et al. Adjacent Fe Site boosts electrocatalytic oxygen evolution at Co site in single-atom-catalyst through a dual-metal-site design[J]. Energy & Environmental Science, 2023, 16(4): 1685-1696. [4] YAO Y, LIAO G, CUI H, et al. Monodispersed In2O3@ZIF-8 core-shell nanocomposite with selectivity and enhanced photocatalytic activity[J]. Journal of Materials Science: Materials in Electronics, 2023, 34(18): 1445. doi: 10.1007/s10854-023-10870-4 [5] YANG X, LI Q X, CHI S Y, et al. Hydrophobic perfluoroalkane modified metal-organic frameworks for the enhanced electrocatalytic reduction of CO2[J]. SmartMat, 2022, 3(1): 163-172. doi: 10.1002/smm2.1086 [6] ZHU W, SONG X, LIAO F, et al. Stable and oxidative charged Ru enhance the acidic oxygen evolution reaction activity in two-dimensional ruthenium-iridium oxide[J]. Nat Commun, 2023, 14(1): 5365. doi: 10.1038/s41467-023-41036-9 [7] SONG F, LI W, YANG J, et al. Interfacing nickel nitride and nickel boosts both electrocatalytic hydrogen evolution and oxidation reactions[J]. Nat Commun, 2018, 9(1): 4531. doi: 10.1038/s41467-018-06728-7 [8] YAO M, WANG B, SUN B, et al. Rational design of self-supported Cu@WC core-shell mesoporous nanowires for pH-universal hydrogen evolution reaction[J]. Applied Catalysis B: Environmental, 2021, 280: 119451. doi: 10.1016/j.apcatb.2020.119451 [9] INAMDAR A I, CHAVAN H S, HOU B, et al. A Robust Nonprecious CuFe Composite as a Highly Efficient Bifunctional Catalyst for Overall Electrochemical Water Splitting[J]. Small, 2020, 16(2): e1905884. doi: 10.1002/smll.201905884 [10] LI L, WANG B, ZHANG G, et al. Electrochemically Modifying the Electronic Structure of IrO2 Nanoparticles for Overall Electrochemical Water Splitting with Extensive Adaptability[J]. Advanced Energy Materials, 2020, 10(30): 2001600. doi: 10.1002/aenm.202001600 [11] YU L, WU L, MCELHENNY B, et al. Ultrafast room-temperature synthesis of porous S-doped Ni/Fe (oxy)hydroxide electrodes for oxygen evolution catalysis in seawater splitting[J]. Energy & Environmental Science, 2020, 13(10): 3439-3446. [12] WANG P, PU Z, LI W, et al. Coupling NiSe2-Ni2P heterostructure nanowrinkles for highly efficient overall water splitting[J]. Journal of Catalysis, 2019, 377: 600-608. doi: 10.1016/j.jcat.2019.08.005 [13] GONG Y N, JIAO L, QIAN Y, et al. Regulating the Coordination Environment of MOF-Templated Single-Atom Nickel Electrocatalysts for Boosting CO2 Reduction[J]. Angewandte Chemie International Edition, 2020, 59(7): 2705-2709. doi: 10.1002/anie.201914977 [14] ZHANG Y, HAN Y, DENG F, et al. Enhancement of the performance of Ge-air batteries under high temperatures using conductive MOF-modified Ge anodes[J]. Carbon Energy, 2024: e580. [15] MADHU R, KARMAKAR A, BERA K, et al. Recent developments in transition metal-based MOFs for electrocatalytic water splitting emphasizing fundamental and structural aspects[J]. Materials Chemistry Frontiers, 2023, 7(11): 2120-2152. doi: 10.1039/D3QM00089C [16] LIM Y, KIM B, KIM J. Data-Driven Design of Flexible Metal-Organic Frameworks for Gas Storage[J]. Chemistry of Materials, 2024, 36(11): 5465-5473. doi: 10.1021/acs.chemmater.4c00398 [17] YU M-H, GENG L, CHANG Z, et al. Coordination Bonding Directed Molecular Assembly toward Functional Metal-Organic Frameworks: From Structural Regulation to Properties Modulation[J]. Accounts of Materials Research, 2023, 4(10): 839-853. doi: 10.1021/accountsmr.3c00097 [18] DONG P, ZHANG X, HISCOX W, et al. Toward High-Performance Metal-Organic-Framework-Based Quasi-Solid-State Electrolytes: Tunable Structures and Electrochemical Properties[J]. Adv Mater, 2023, 35(32): e2211841. doi: 10.1002/adma.202211841 [19] XUE Z, LIU K, LIU Q, et al. Missing-linker metal-organic frameworks for oxygen evolution reaction[J]. Nat Commun, 2019, 10(1): 5048. doi: 10.1038/s41467-019-13051-2 [20] CHENG W. Lattice-strained metal-organic-framework arrays for bifunctional oxygen electrocatalysis[J]. Nat. Energy, 2019, 4(2): 115-122. doi: 10.1038/s41560-018-0308-8 [21] MA Y, LENG D, ZHANG X, et al. Enhanced Activities in Alkaline Hydrogen and Oxygen Evolution Reactions on MoS2 Electrocatalysts by In-Plane Sulfur Defects Coupled with Transition Metal Doping[J]. Small, 2022, 18(39): 2203173. doi: 10.1002/smll.202203173 [22] HUANG Y, HAN J, WANG H, et al. Multiple metallic dopants in nickel nanoparticles for electrocatalytic oxygen evolution[J]. Progress in Natural Science: Materials International, 2023, 33(1): 67-73. doi: 10.1016/j.pnsc.2023.03.002 [23] ZHANG J, CHENG C, XIAO L, et al. Construction of Co-Se-W at Interfaces of Phase-Mixed Cobalt Selenide via Spontaneous Phase Transition for Platinum-Like Hydrogen Evolution Activity and Long-Term Durability in Alkaline and Acidic Media[J]. Adv Mater, 2024, 36(28): e2401880. doi: 10.1002/adma.202401880 [24] SUN P, QIAO Z, DONG X, et al. Designing 3d Transition Metal Cation-Doped MRuO(x) As Durable Acidic Oxygen Evolution Electrocatalysts for PEM Water Electrolyzers[J]. J Am Chem Soc, 2024, 146(22): 15515-15524. doi: 10.1021/jacs.4c04096 [25] LIU X, WEI S, CAO S, et al. Lattice Strain with Stabilized Oxygen Vacancies Boosts Ceria for Robust Alkaline Hydrogen Evolution Outperforming Benchmark Pt[J]. Adv Mater, 2024, 36(33): e2405970. doi: 10.1002/adma.202405970 [26] Zhou L, Shao Y, Yin F, et al. Stabilizing non-iridium active sites by non-stoichiometric oxide for acidic water oxidation at high current density[J]. Nature Communications, 2023, 14(1): 7644. doi: 10.1038/s41467-023-43466-x [27] CHEN X, XU X, CHENG Y, et al. Achieving High-Performance Electrocatalytic Water Oxidation on Ni(OH)(2) with Optimized Intermediate Binding Energy Enabled by S-Doping and CeO(2) -Interfacing[J]. Small, 2024, 20(8): e2303169. doi: 10.1002/smll.202303169 [28] GUO X, WAN X, LIU Q, et al. Phosphated IrMo bimetallic cluster for efficient hydrogen evolution reaction[J]. eScience, 2022, 2(3): 304-310. doi: 10.1016/j.esci.2022.04.002 [29] QI Y, XIAO X, MEI Y, et al. Modulation of Brønsted and Lewis Acid Centers for NixCo3-xO4 Spinel Catalysts: Towards Efficient Catalytic Conversion of Lignin[J]. Advanced Functional Materials, 2022, 32(15): 2111615. doi: 10.1002/adfm.202111615 [30] ZHOU J, QIAO F, REN Z, et al. Amorphization Engineering of Bimetallic Metal-Organic Frameworks to Identify Volcano-Type Trend toward Oxygen Evolution Reaction[J]. Advanced Functional Materials, 2023, 34(1): 2304380. [31] ZHAO S, WANG Y, DONG J, et al. Ultrathin metal-organic framework nanosheets for electrocatalytic oxygen evolution[J]. Nature Energy, 2016, 1(12): 1-10. [32] HUANG L, GAO G, ZHANG H, et al. Self-dissociation-assembly of ultrathin metal-organic framework nanosheet arrays for efficient oxygen evolution[J]. Nano Energy, 2020, 68: 104296. doi: 10.1016/j.nanoen.2019.104296 [33] SUN F, WANG G, DING Y, et al. NiFe-Based Metal-Organic Framework Nanosheets Directly Supported on Nickel Foam Acting as Robust Electrodes for Electrochemical Oxygen Evolution Reaction[J]. Advanced Energy Materials, 2018, 8(21): 1800584. doi: 10.1002/aenm.201800584 [34] BORDIGA S, LAMBERTI C, RICCHIARDI G, et al. Electronic and vibrational properties of a MOF-5 metal-organic framework: ZnO quantum dot behaviour[J]. Chem Commun (Camb), 2004, (20): 2300-1. doi: 10.1039/B407246D [35] WANG C-P, FENG Y, SUN H, et al. Self-Optimized Metal-Organic Framework Electrocatalysts with Structural Stability and High Current Tolerance for Water Oxidation[J]. ACS Catalysis, 2021, 11(12): 7132-7143. doi: 10.1021/acscatal.1c01447 [36] CHEN G, WANG T, ZHANG J, et al. Accelerated Hydrogen Evolution Kinetics on NiFe-Layered Double Hydroxide Electrocatalysts by Tailoring Water Dissociation Active Sites[J]. Adv Mater, 2018, 30(10): 1706279. doi: 10.1002/adma.201706279 [37] ZHANG L-N, LANG Z-L, WANG Y-H, et al. Cable-like Ru/WNO@C nanowires for simultaneous high-efficiency hydrogen evolution and low-energy consumption chlor-alkali electrolysis[J]. Energy & Environmental Science, 2019, 12(8): 2569-2580. [38] PENG Y, LU B, CHEN L, et al. Hydrogen evolution reaction catalyzed by ruthenium ion-complexed graphitic carbon nitride nanosheets[J]. Journal of Materials Chemistry A, 2017, 5(34): 18261-18269. doi: 10.1039/C7TA03826G [39] LU B, GUO L, WU F, et al. Ruthenium atomically dispersed in carbon outperforms platinum toward hydrogen evolution in alkaline media[J]. Nat Commun, 2019, 10(1): 631. doi: 10.1038/s41467-019-08419-3 [40] WANG Z-L, SUN K, HENZIE J, et al. Spatially Confined Assembly of Monodisperse Ruthenium Nanoclusters in a Hierarchically Ordered Carbon Electrode for Efficient Hydrogen Evolution[J]. Angewandte Chemie International Edition, 2018, 57(20): 5848-5852. doi: 10.1002/anie.201801467 [41] LI W, ZHANG H, ZHANG K, et al. Monodispersed ruthenium nanoparticles interfacially bonded with defective nitrogen-and-phosphorus-doped carbon nanosheets enable pH-universal hydrogen evolution reaction[J]. Applied Catalysis B: Environmental, 2022, 306: 121095. doi: 10.1016/j.apcatb.2022.121095 [42] LI W, ZHANG H, HONG M, et al. Defective RuO2/TiO2 nano-heterostructure advances hydrogen production by electrochemical water splitting[J]. Chemical Engineering Journal, 2022, 431: 134072. doi: 10.1016/j.cej.2021.134072 [43] ZHANG M, CHEN J, LI H, et al. Ru-RuO2/CNT hybrids as high-activity pH-universal electrocatalysts for water splitting within 0.73 V in an asymmetric-electrolyte electrolyzer[J]. Nano Energy, 2019, 61: 576-583. doi: 10.1016/j.nanoen.2019.04.050 [44] FAN R, MU Q, WEI Z, et al. Atomic Ir-doped NiCo layered double hydroxide as a bifunctional electrocatalyst for highly efficient and durable water splitting[J]. Journal of Materials Chemistry A, 2020, 8(19): 9871-9881. doi: 10.1039/D0TA03272G [45] LI Z, WU Y, LU G. Highly efficient hydrogen evolution over Co(OH)2 nanoparticles modified g-C3N4 co-sensitized by Eosin Y and Rose Bengal under Visible Light Irradiation[J]. Applied Catalysis B: Environmental, 2016, 188: 56-64. doi: 10.1016/j.apcatb.2016.01.057 [46] LI G, LI J, LIU X, et al. Ir-doped Co-BDC MOF as efficient bifunctional catalyst for overall electrochemical water splitting[J]. Ionics, 2023, 29(5): 1963-1973. doi: 10.1007/s11581-023-04928-w [47] SUN Y, XUE Z, LIU Q, et al. Modulating electronic structure of metal-organic frameworks by introducing atomically dispersed Ru for efficient hydrogen evolution[J]. Nat Commun, 2021, 12(1): 1369. doi: 10.1038/s41467-021-21595-5 [48] CAO C, MA D D, XU Q, et al. Semisacrificial Template Growth of Self-Supporting MOF Nanocomposite Electrode for Efficient Electrocatalytic Water Oxidation[J]. Advanced Functional Materials, 2018, 29(6): 1970033. [49] WANG Y, ZHU R, WANG Z, et al. Cu induced formation of dendritic CoFeCu ternary alloys on Ni foam for efficient oxygen evolution reaction[J]. Journal of Alloys and Compounds, 2021, 880: 160523. doi: 10.1016/j.jallcom.2021.160523 [50] LI W, ZHANG H, ZHANG K, et al. Altered electronic structure of trimetallic FeNiCo-MOF nanosheets for efficient oxygen evolution[J]. Chem Commun (Camb), 2023, 59(32): 4750-4753. doi: 10.1039/D2CC06727G [51] WANG P, ZHU J, PU Z, et al. Interfacial engineering of Co nanoparticles/Co2C nanowires boosts overall water splitting kinetics[J]. Applied Catalysis B: Environmental, 2021, 296: 120334. doi: 10.1016/j.apcatb.2021.120334 [52] WANG R, SUN X, ZHONG J, et al. Low-temperature plasma-assisted synthesis of iron and nitrogen co-doped CoFeP-N nanowires for high-efficiency electrocatalytic water splitting[J]. Applied Catalysis B: Environment and Energy, 2024, 352: 124027. doi: 10.1016/j.apcatb.2024.124027 [53] ZHANG Z, WANG H, LI Y, et al. Confined Pyrolysis Synthesis of Well-dispersed Cobalt Copper Bimetallic Three-dimensional N-Doped Carbon Framework as Efficient Water Splitting Electrocatalyst[J]. Chemical Research in Chinese Universities, 2022, 38(3): 750-757. doi: 10.1007/s40242-022-1504-4 [54] GUO T, CHEN L, LI Y, et al. Controllable Synthesis of Ultrathin Defect-Rich LDH Nanoarrays Coupled with MOF-Derived Co-NC Microarrays for Efficient Overall Water Splitting[J]. Small, 2022, 18(29): e2107739. doi: 10.1002/smll.202107739 -
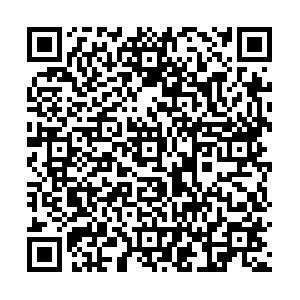
计量
- 文章访问数: 30
- HTML全文浏览量: 15
- 被引次数: 0