Research progress for single component fuel cell
-
摘要: 传统固体氧化物燃料电池(SOFC)需要保持较高的工作温度,不利于其不同组分的兼容和长期稳定性,这阻碍了SOFC的商业化进展。若降低反应温度则会带来显著的界面阻力和反应动力学损失,使得输出功率降低。最近,单部件燃料电池(SLFC)作为一种新型能源转换装置被提出,与传统三组分SOFC不同,SLFC的特点是具有一个半导体–离子异质结构材料混合离子导电的均匀层,p-n异质结构和内建电场的存在可以实现电荷分离,提高了燃料电池的稳定性和耐久性,使其在低温下也具备良好的离子电导和电池性能,具有广阔的发展前景。本文对最近几年以来SLFC领域的研究进展做了一个简要的综述,回顾了SLFC中异质结与能带对准隔绝电子的工作原理,研究空间电荷区与晶格应变对界面离子传导的影响,总结了研究者在半导体-离子材料上做出的改进,并讨论了该燃料电池的优势和未来的发展方向。Abstract: Traditional solid oxide fuel cells (SOFCs) require high operating temperatures, which are not conducive to the compatibility and long-term stability of their various components, hindering the commercial progress of SOFCs. Reducing the reaction temperature can lead to significant interfacial resistance and losses in reaction kinetics, resulting in a reduced output power. Recently, single-component fuel cell (SLFC) has been proposed as a new type of energy conversion device. Unlike the traditional three-component SOFCs, the SLFC is characterized by a homogeneous layer of semiconductor-ion heterostructure material with mixed ionic conductivity. The presence of p-n heterojunctions and built-in electric fields can achieve charge separation, enhancing the stability and durability of the fuel cell, allowing it to have good ionic conductivity and cell performance even at low temperatures, and offering broad prospects for development. This paper provides a brief overview of the research progress in the field of SLFCs in recent years, reviewing the working principle of heterojunctions and energy band alignment in SLFCs to isolate electrons, studying the effects of space charge regions and lattice strain on interfacial ionic conduction, summarizing the improvements made by researchers on semiconductor-ionic materials, and discussing the advantages and future development directions of SLFC.
-
Key words:
- single component fuel cell /
- p-n heterojunction /
- ionic conductor /
- perovskite /
- semiconductor material
-
图 4 (a) CeO2中导电率增强的对数与晶格应变的关系图[29];(b) SDC和Sr0.92Ti0.5Fe0.5O3–δ物质间的受力示意图和两者晶体结构[30];(c) 不同模型过度路径中掺杂–质子相互作用能、相应的最大氧位移和最大质子跃迁能;(d) 显示质子与O2–在纯BaZrO3和在一个Y掺杂环境中的结合能与晶格畸变;(e) 掺杂剂–质子相互作用能的最大氧位移的函数图[28]
Figure 4. (a) plot of the logarithm of ionic conductivity enhancement in CeO2 versus lattice strain[29]; (b) Schematic representation of the forces between SDC and Sr0.92Ti0.5Fe0.5O3–δ substances and their crystal structures[30]; (c) The dopant-proton interaction energy (representing protonated samples), the corresponding maximum oxygen displacement and maximum proton transition energy among the various transition pathways for different model systems; (d) A schematic showing the correlation between proton binding energy and O2– sublattice distortion for various local environments such as pure BaZrO3, in one yttrium dopant environment; (e) The dopant-proton interaction energy plotted as a function of maximum oxygen displacement[28]
图 5 (a) CeO2–δ中氧空位形成时电子结构变化示意图;(b) CeO2–δ-CeO2颗粒界面处的电荷分离;(c) 质子被限制在粒子表面[36]
Figure 5. (a) Schematic representation of the change in the electronic structure during the formation of oxygen vacancies in CeO2–δ; (b) Charge separation at the interface of the CeO2–δ-CeO2 particles; (c) Proton confinement to the surface of the particle[36]
图 9 (a) 空气和 (b) H2/空气气氛下550℃ Ni-NCAL/CZO-SDC的整流效果;(c) 异质结构中的电荷输运示意图[51];(d) 8 GDC-2 NCO复合材料H+/O2–和H+的输运机制;(e) 8 GDC-2 NCO复合电解质燃料电池在不同温度下的I-V和I-P特性曲线[52]
Figure 9. rectification effect of Ni-NCAL/CZO-SDC at 550℃ in (a)air and (b) H2/air atmospheres; (c) Schematic of charge transport in the heterostructures[51]; (d) 8 GDC-2 NCO composite H+/O2– and H+ transport mechanisms; (e) I-V and I-P characteristic curves of 8 GDC-2 NCO composite electrolyte fuel cell at different temperatures[52]
表 1 近五年SLFC研究进展
Table 1. Progress of SLFC research in the last five years
Configuration T/oC OCV/V Pmax /(W·cm–2) Ref. NCAL/ Pr0.4Sr0.6Co0.2Fe0.7Nb0.1O3−δ-LNSDC /NCAL 550 0.98 0.24 [50] Ni-NCAL/CeO2/NCAL-Ni 520 1.08 0.70 [36] Ni-NCAL/BaCo0.4Fe0.4Zr0.1Y0.1O3–δ-ZnO/NCAL-Ni 100-500 1.01-1.08 0.25-0.64 [58] Ni-NCAL/Ce0.8Sm0.2O1.9 -La1.85Sr0.15CuO4/NCAL-Ni 550 1.0 0.90 [56] NCAL-Ni/7 Ba0.5Sr0.5Co0.4Fe0.4Zr0.1Y0.1O3–δ-3 Ca0.04Ce0.80Sm0.16O2−δ/NCAL-Ni 520 1.07 0.90 [59] Ni-NCAL/Co0.2Zn0.8O -SDC/NCAL-Ni 520 1.07 0.93 [51] Ni-NCAL/BaCo0.2Fe0.1Ce0.2Tm0.1Zr0.3Y0.1O3–δ/NCAL-Ni 530 1.09 0.87 [60] Ni-NCAL/SDC-SrTiO3/NCAL-Ni 550 1.1 0.89 [47] Ni-NCAL/La0.5Ba0.5Co0.2Fe0.2Zr0.3Y0.3O3–δ/NCAL-Ni 450-550 1.09-1.1 0.29-0.66 [61] Ni-NCAL/Li2TiO3-LaSrCoFeO3/NCAL-Ni 550 1.09 0.35 [62] Ag/7 LNSDC-3 Pr0.4Sr0.5Fe0.9Mo0.1O3/Ag 700 ~1.0 0.33 [63] NCAL-Ni/SrFe0.3Ti0.8O3-WO3/NCAL-Ni 520 1.04 0.88 [64] Ni-NCAL/8 GDC-2 NaCoO2/NCAL-Ni 550 1.06 1.10 [52] NCAL/r-La0.2Sr0.7Ti0.9Ni0.1O3−δ-LNSDC/NCAL 550 1.13 0.65 [46] Ni-NCAL/La0.8Sr0.2Co0.8Fe0.2O3-GDC/NCAL-Ni 550 1.1 1.06 [65] Ni-NCAL/Fe0.1Gd1.9O3/NCAL-Ni 550 1.1 1.35 [66] Ni-NCAL/Co dopedY2O3/Ni-NCAL 530 1.09 0.86 [67] Ni-NCAL/La0.8Sr0.2Co0.8Fe0.2O3–δ-CeO2/NCAL-Ni 520 ~1.0 0.50 [68] Ni-NCAL/3 CuFeO2-7 ZnO/NCAL-Ni 550 1.06 0.56 [69] Notes:NCAL is Ni0.8Co0.15Al0.05LiO2; LNSDC is Sm0.2Ce0.8O2-(Li/Na)2CO3; SDC is Ce0.8Sm0.2O1.9; GDC is Ce0.8Gd0.2O1.9; r is reduced; is test temperature; OCV is open circuit voltage; Pmax is peak power density. -
[1] ZHAI S, ZHAO R, LIAO H, et al. Enhancing layered perovskite ferrites with ultra-high-density nanoparticles via cobalt doping for ceramic fuel cell anode[J]. Journal of Energy Chemistry, 2024, 96: 39-48. doi: 10.1016/j.jechem.2024.04.011 [2] ZHANG Z, CHEN S, ZHANG H, et al. In situ self-assembled NdBa0.5Sr0.5Co2O5+δ/Gd0.1Ce0.9O2–δ hetero-interfaces enable enhanced electrochemical activity and CO2 durability for solid oxide fuel cells[J]. Journal of Colloid and Interface Science, 2024, 655: 157-166. doi: 10.1016/j.jcis.2023.11.009 [3] WANG H, ZHU W, XU L, et al. Self-assembled nanocomposite based on SrCo0.7Fe0.2Sc0.1O3−δ as an efficient intermediate-to-Low-temperature SOFC cathode[J]. ACS Applied Materials & Interfaces, 2024, 16(27): 34988-34996. [4] HAN X, LING Y, YANG Y, et al. Utilizing high entropy effects for developing chromium-tolerance cobalt-free cathode for solid oxide fuel cells[J]. Advanced Functional Materials, 2023, 33(43): 2304728. doi: 10.1002/adfm.202304728 [5] ZHANG L, JIANG Y, ZHU K, et al. Fe-doped SDC solid solution as an electrolyte for low-to-intermediate-temperature solid oxide fuel cells[J]. ACS Applied Materials & Interfaces, 2024, 16(4): 4648-4660. [6] LIU D, ZHU H, YUAN S, et al. Understanding the oxygen-vacancy-related catalytic cycle for H2 oxidation on ceria-based SOFC anode and the promotion effect of lanthanide doping from theoretical perspectives[J]. Applied Surface Science, 2022, 576: 151803. doi: 10.1016/j.apsusc.2021.151803 [7] KATTA V S, DAS A, DILEEP K R, et al. Vacancies induced enhancement in neodymium doped titania photoanodes based sensitized solar cells and photo-electrochemical cells[J]. Solar Energy Materials and Solar Cells, 2021, 220: 110843. doi: 10.1016/j.solmat.2020.110843 [8] ZHU B, RAZA R, ABBAS G, et al. An electrolyte-free fuel cell constructed from one homogenous layer with mixed conductivity[J]. Advanced Functional Materials, 2011, 21(13): 2465-2469. doi: 10.1002/adfm.201002471 [9] ZHU B, YUN S, LUND P D. Semiconductor-ionic materials could play an important role in advanced fuel-to-electricity conversion[J]. International Journal of Energy Research, 2018, 42(11): 3413-3415. doi: 10.1002/er.4105 [10] SHAH M A K Y, TAYYAB Z, RAUF S, et al. Interface engineering of bi-layer semiconductor SrCoSnO3– δ-CeO2– δ heterojunction electrolyte for boosting the electrochemical performance of low-temperature ceramic fuel cell[J]. International Journal of Hydrogen Energy, 2021, 46(68): 33969-33977. doi: 10.1016/j.ijhydene.2021.07.204 [11] SINGH K, NOWOTNY J, THANGADURAI V. Amphoteric oxide semiconductors for energy conversion devices: a tutorial review[J]. Chemical Society Reviews, 2013, 42(5): 1961-1972. doi: 10.1039/C2CS35393H [12] OIKAWA T, OHDAIRA K, HIGASHIMINE K, et al. Application of crystalline silicon surface oxidation to silicon heterojunction solar cells[J]. Current Applied Physics, 2015, 15(10): 1168-1172. doi: 10.1016/j.cap.2015.07.004 [13] SHAH M A K Y, LU Y, MUSHTAQ N, et al. Semiconductor-membrane fuel cell (SMFC) for renewable energy technology[J]. Renewable and Sustainable Energy Reviews, 2023, 185: 113639. doi: 10.1016/j.rser.2023.113639 [14] LI C, DONG S, TANG R, et al. Heteroatomic interface engineering in MOF-derived carbon heterostructures with built-in electric-field effects for high performance Al-ion batteries[J]. Energy & Environmental Science, 2018, 11(11): 3201-3211. [15] LALWANI S, ALNAHYAN M, AL ZAABI A, et al. Advances in interfacial engineering and their role in heterostructure formation for HER applications in wider pH[J]. ACS Applied Energy Materials, 2022, 5(12): 14571-14592. doi: 10.1021/acsaem.2c02102 [16] LU Y, ZHU B, SHI J, et al. Advanced low-temperature solid oxide fuel cells based on a built-in electric field[J]. Energy Materials, 2021, 1(1): 100007. [17] HU E, JIANG Z, FAN L, et al. Junction and energy band on novel semiconductor-based fuel cells[J]. iScience, 2021, 24(3): 102191. doi: 10.1016/j.isci.2021.102191 [18] ZHANG Y, LIU J, SINGH M, et al. Superionic conductivity in ceria-Based heterostructure composites for low-temperature solid oxide fuel cells[J]. Nano-Micro Letters, 2020, 12(1): 178. doi: 10.1007/s40820-020-00518-x [19] HU M, CHEN M, WANG Y, et al. A p-n heterostructure composite of NaCrO2 and CeO2 for intermediate temperature solid oxide fuel cells[J]. Journal of Alloys and Compounds, 2023, 962: 171169. doi: 10.1016/j.jallcom.2023.171169 [20] LI Y, ZHENG N, YU L, et al. A simple phenyl group introduced at the tail of alkyl side chains of small molecular acceptors: new strategy to balance the crystallinity of acceptors and miscibility of bulk heterojunction enabling highly efficient organic solar cells[J]. Advanced Materials, 2019, 31(12): 1807832. doi: 10.1002/adma.201807832 [21] ZHU B, MI Y, XIA C, et al. A nanoscale perspective on solid oxide and semiconductor membrane fuel cells: materials and technology[J]. Energy Materials, 2021, 1(1): 100002. [22] FABBRI E, PERGOLESI D, TRAVERSA E. Ionic conductivity in oxide heterostructures: the role of interfaces[J]. Science and Technology of Advanced Materials, 2010, 11(5): 054503. doi: 10.1088/1468-6996/11/5/054503 [23] MAIER J. Ionic transport in nano-sized systems[J]. Solid State Ionics, 2004, 175(1): 7-12. [24] GUO X, WASER R. Electrical properties of the grain boundaries of oxygen ion conductors: Acceptor-doped zirconia and ceria[J]. Progress in Materials Science, 2006, 51(2): 151-210. doi: 10.1016/j.pmatsci.2005.07.001 [25] WANG L, XIE R, CHEN B, et al. In-situ visualization of the space-charge-layer effect on interfacial lithium-ion transport in all-solid-state batteries[J]. Nature Communications, 2020, 11(1): 5889. doi: 10.1038/s41467-020-19726-5 [26] SINGH M, SINGH A K. Space charge layer induced superionic conduction and charge transport behaviour of “alkali carbonates and tri-doped ceria nanocomposites” for LT-SOFCs applications[J]. Ceramics International, 2021, 47(1): 1218-1228. doi: 10.1016/j.ceramint.2020.08.241 [27] UTHAYAKUMAR A, PANDIYAN A, MATHIYALAGAN S, et al. The effect of space charge on blocking grain boundary resistance in an yttrium-doped barium zirconate electrolyte for solid oxide fuel cells[J]. The Journal of Physical Chemistry C, 2020, 124(10): 5591-5599. doi: 10.1021/acs.jpcc.0c00166 [28] DING J, BALACHANDRAN J, SANG X, et al. The influence of local distortions on proton mobility in acceptor doped perovskites[J]. Chemistry of Materials, 2018, 30(15): 4919-4925. doi: 10.1021/acs.chemmater.8b00502 [29] WEN K, ZHANG K H L, WANG W, et al. Physical justification for ionic conductivity enhancement at strained coherent interfaces[J]. Journal of Power Sources, 2015, 285: 37-42. doi: 10.1016/j.jpowsour.2015.02.089 [30] WANG J, JIANG Q, LIU D, et al. Effect of inner strain on the performance of dual-phase oxygen permeable membranes[J]. Journal of Membrane Science, 2022, 644: 120142. doi: 10.1016/j.memsci.2021.120142 [31] TIAN A, MEI Z, WANG L, et al. Improved photocatalytic carbon dioxide reduction over Bi-doped CeO2 by strain engineering[J]. Sustainable Energy & Fuels, 2024, 8(7): 1405-1411. [32] YANG G, EL LOUBANI M, CHALAKI H R, et al. Tuning ionic conductivity in fluorite Gd-doped CeO2-bixbyite RE2O3 (RE = Y and Sm) multilayer thin films by controlling interfacial strain[J]. ACS Applied Electronic Materials, 2023, 5(8): 4556-4563. doi: 10.1021/acsaelm.3c00724 [33] MOHAN KANT K, ESPOSITO V, PRYDS N. Strain induced ionic conductivity enhancement in epitaxial Ce0.9Gd0.1O2– δ thin films[J]. Applied Physics Letters, 2012, 100(3): 033105. doi: 10.1063/1.3676659 [34] MAYESHIBA T, MORGAN D. Strain effects on oxygen migration in perovskites[J]. Physical Chemistry Chemical Physics, 2015, 17(4): 2715-2721. doi: 10.1039/C4CP05554C [35] CHEN G, LIU H, HE Y, et al. Electrochemical mechanisms of an advanced low-temperature fuel cell with a SrTiO3 electrolyte[J]. Journal of Materials Chemistry A, 2019, 7(16): 9638-9645. doi: 10.1039/C9TA00499H [36] XING Y, WU Y, LI L, et al. Proton shuttles in CeO2/CeO2– δ core-Shell structure[J]. ACS Energy Letters, 2019, 4(11): 2601-2607. doi: 10.1021/acsenergylett.9b01829 [37] LIU Y, MUSHTAQ M N, ZHANG W, et al. Single-phase electronic-ionic conducting Sm3+/Pr3+/Nd3+ triple-doped ceria for new generation fuel cell technology[J]. International Journal of Hydrogen Energy, 2018, 43(28): 12817-12824. doi: 10.1016/j.ijhydene.2018.04.125 [38] LI J, XIE J, LI D, et al. An Interface heterostructure of NiO and CeO2 for using electrolytes of low-temperature solid oxide fuel cells[J]. 2021, 11(8): 2004. [39] ZHU W, XIA C, DING D, et al. Electrical properties of ceria-carbonate composite electrolytes[J]. Materials Research Bulletin, 2006, 41(11): 2057-2064. doi: 10.1016/j.materresbull.2006.04.001 [40] GöBEL M C, GREGORI G, MAIER J. Numerical calculations of space charge layer effects in nanocrystalline ceria. Part II: detailed analysis of the space charge layer properties[J]. Physical Chemistry Chemical Physics, 2014, 16(21): 10175-10186. doi: 10.1039/C3CP54616K [41] WANG B, WANG Y, FAN L, et al. Preparation and characterization of Sm and Ca co-doped ceria-La0.6Sr0.4Co0.2Fe0.8O3– δ semiconductor-ionic composites for electrolyte-layer-free fuel cells[J]. Journal of Materials Chemistry A, 2016, 4(40): 15426-15436. doi: 10.1039/C6TA05763B [42] RAZA R, KHAN A, RAFIQUE A, et al. Nanocomposite BaZr0.7Sm0.1Y0.2O3– δ-La0.8Sr0.2Co0.2Fe0.8O3– δ materials for single layer fuel cell[J]. International Journal of Hydrogen Energy, 2017, 42(34): 22280-22287. doi: 10.1016/j.ijhydene.2017.04.287 [43] LI P, LIU F, YANG B, et al. Enhanced electrochemical redox kinetics of La0.6Sr0.4Co0.2Fe0.8O3 in reversible solid oxide cells[J]. Electrochimica Acta, 2023, 446: 142069. doi: 10.1016/j.electacta.2023.142069 [44] MIRUSZEWSKI T, GDANIEC P, ROSIŃSKI W, et al. Structure and electrical properties of Y, Fe-based perovskite mixed conducting composites fabricated by a modified polymer precursor method[J]. Solid State Sciences, 2017, 70: 41-46. doi: 10.1016/j.solidstatesciences.2017.06.008 [45] GAO J, XU S, AKBAR M, et al. Single layer low-temperature SOFC based on Ce0.8Sm0.2O2– δ-La0.25Sr0.75Ti1 - Ni0.8Co0.15Al0.05LiO2– δ composite material[J]. International Journal of Hydrogen Energy, 2021, 46(15): 9775-9781. doi: 10.1016/j.ijhydene.2020.07.043 [46] WANG Z, MENG Y, SINGH M, et al. Ni/NiO exsolved perovskite La0.2Sr0.7Ti0.9Ni0.1O3– δ for semiconductor-Ionic fuel cells: Roles of Electrocatalytic Activity and Physical Junctions[J]. ACS Applied Materials & Interfaces, 2023, 15(1): 870-881. [47] CAI Y, CHEN Y, AKBAR M, et al. A bulk-heterostructure nanocomposite electrolyte of Ce0.8Sm0.2O2– δ-SrTiO3 for low-temperature solid oxide fuel cells[J]. Nano-Micro Letters, 2021, 13(1): 46. doi: 10.1007/s40820-020-00574-3 [48] MENG Y, AKBAR M, GAO J, et al. Superionic conduction of self-assembled heterostructural LSCrF-CeO2 electrolyte for solid oxide fuel cell at 375-550℃[J]. Applied Surface Science, 2024, 645: 158832. doi: 10.1016/j.apsusc.2023.158832 [49] LIU Y, TANG Y, Ma Z, et al. Flowerlike CeO2 microspheres coated with Sr2Fe1.5Mo0.5Ox nanoparticles for an advanced fuel cell[J]. Scientific Reports, 2015, 5(1): 11946. doi: 10.1038/srep11946 [50] SHAO K, LI F, ZHANG G, et al. Approaching durable single-layer fuel cells: promotion of electroactivity and charge separation via nanoalloy redox exsolution[J]. ACS Applied Materials & Interfaces, 2019, 11(31): 27924-27933. [51] RAUF S, SHAH M A K Y, ZHU B, et al. Electrochemical properties of a dual-ion semiconductor-ionic Co0.2Zn0.8O-Sm0.20Ce0.80O2– δ composite for a high-performance low-temperature solid oxide fuel cell[J]. ACS Applied Energy Materials, 2021, 4(1): 194-207. doi: 10.1021/acsaem.0c02095 [52] YU Y, CHENG X, KHALID M A, et al. Gadolinium-Doped Ceria-NaCoO2 heterogeneous semiconductor ionic materials for solid oxide fuel cell Application[J]. ACS Applied Energy Materials, 2023, 6(18): 9508-9515. doi: 10.1021/acsaem.3c01487 [53] CAI Y, WANG B, WANG Y, et al. Validating the technological feasibility of yttria-stabilized zirconia-based semiconducting-ionic composite in intermediate-temperature solid oxide fuel cells[J]. Journal of Power Sources, 2018, 384: 318-327. doi: 10.1016/j.jpowsour.2018.03.012 [54] WANG B, CAI Y, XIA C, et al. Semiconductor-ionic membrane of LaSrCoFe-oxide-doped ceria solid oxide fuel cells[J]. Electrochimica Acta, 2017, 248: 496-504. doi: 10.1016/j.electacta.2017.07.128 [55] ZHU B, HUANG Y, FAN L, et al. Novel fuel cell with nanocomposite functional layer designed by perovskite solar cell principle[J]. Nano Energy, 2016, 19: 156-164. doi: 10.1016/j.nanoen.2015.11.015 [56] YUAN M, DONG W, WEI L, et al. Stability study of SOFC using layered perovskite oxide La1.85Sr0.15CuO4 mixed with ionic conductor as membrane[J]. Electrochimica Acta, 2020, 332: 135487. doi: 10.1016/j.electacta.2019.135487 [57] HU H, LIN Q, ZHU Z, et al. Time-dependent performance change of single layer fuel cell with Li0.4Mg0.3Zn0.3O/Ce0.8Sm0.2O2– δ composite[J]. International Journal of Hydrogen Energy, 2014, 39(20): 10718-10723. doi: 10.1016/j.ijhydene.2014.04.185 [58] XIA C, MI Y, WANG B, et al. Shaping triple-conducting semiconductor BaCo0.4Fe0.4Zr0.1Y0.1O3– δ into an electrolyte for low-temperature solid oxide fuel cells[J]. Nature Communications, 2019, 10(1): 1707. doi: 10.1038/s41467-019-09532-z [59] RAUF S, ZHU B, YOUSAF SHAH M A K, et al. Application of a triple-conducting heterostructure electrolyte of Ba0.5Sr0.5Co0.1Fe0.7Zr0.1Y0.1O3– δ and Ca0.04Ce0.80Sm0.16O2– δ in a high-performance low-temperature solid oxide fuel cell[J]. ACS Applied Materials & Interfaces, 2020, 12(31): 35071-35080. [60] RAUF S, ZHU B, SHAH M A K Y, et al. Tailoring triple charge conduction in BaCo0.2Fe0.1Ce0.2Tm0.1Zr0.3Y0.1O3– δ semiconductor electrolyte for boosting solid oxide fuel cell performance[J]. Renewable Energy, 2021, 172: 336-349. doi: 10.1016/j.renene.2021.03.031 [61] SHAH M A K Y, RAUF S, MUSHTAQ N, et al. Novel perovskite semiconductor based on Co/Fe-Codoped LBZY (La0.5Ba0.5 Co0.2Fe0.2Zr0.3Y0.3O3– δ) as an electrolyte in ceramic fuel cells[J]. ACS Applied Energy Materials, 2021, 4(6): 5798-5808. doi: 10.1021/acsaem.1c00599 [62] WANG F, XING Y, HU E, et al. Li2TiO3-LaSrCoFeO3 semiconductor heterostructure for low temperature ceramic fuel cell electrolyte[J]. International Journal of Hydrogen Energy, 2021, 46(24): 13265-13272. doi: 10.1016/j.ijhydene.2021.01.174 [63] LI P, YANG P, SHAO T, et al. Evaluating the effect of B-site cation doping on the properties of Pr0.4Sr0.5Fe0.9Mo0.1O3 for reversible single-component cells[J]. Industrial & Engineering Chemistry Research, 2022, 61(15): 5030-5041. [64] SHAH M A K Y, LU Y, MUSHTAQ N, et al. Interfacial active-sites p-n heterojunction SFT-WO3 for enhanced fuel cell performance at 400-500℃[J]. Materials Today Sustainability, 2022, 20: 100229. doi: 10.1016/j.mtsust.2022.100229 [65] ZHAO D, YAN R, MUSHTAQ N, et al. Developing the fast ionic transport in the semiconductor ionic heterostructure composed of La08Sr0.2Co0.8Fe0.2-Gd0.1Ce0.9O2 for the electrolyte application in ceramic fuel cells[J]. Crystals, 2023, 13(4): 697. doi: 10.3390/cryst13040697 [66] LU Y, SHAH M A K Y, MUSHTAQ N, et al. Semiconductor heterostructure (SFT-SnO2) electrolyte with enhanced ionic conduction for ceramic fuel cells[J]. ACS Applied Energy Materials, 2023, 6(12): 6518-6531. doi: 10.1021/acsaem.3c00442 [67] LI J, YOUSAF M, AKBAR M, et al. Processing of high-performance Co doped Y2O3 as a single-phase electrolyte for low temperature solid oxide fuel cell (LT-SOFC)[J]. Ceramics International, 2023, 49(10): 14957-14963. doi: 10.1016/j.ceramint.2022.11.136 [68] XING Y, RAUF S, CAI H, et al. Designing p-n heterostructure of LSC F-CeO2 material for ionic transportation as an electrolyte for semiconductor ion membrane fuel cell[J]. International Journal of Hydrogen Energy, 2024, 50: 428-440. doi: 10.1016/j.ijhydene.2023.08.204 [69] SUI W, JI S, MA X, et al. A dual-layer electrolyte of CuFeO2-ZnO for low-temperature solid oxide fuel cells[J]. International Journal of Hydrogen Energy, 2024, 50: 1126-1136. doi: 10.1016/j.ijhydene.2023.10.067 -
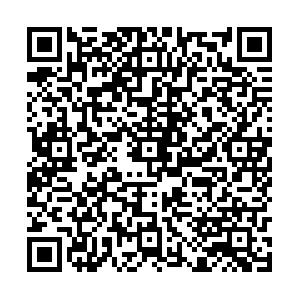
计量
- 文章访问数: 37
- HTML全文浏览量: 27
- 被引次数: 0