Research progress on structural control of radiative cooling materials and its application in buildings
-
摘要: 伴随着化石能源的大量消耗,人类生存环境受到严重威胁。其中,建筑能耗在能源总消耗中所占比例持续攀升,有效管理建筑物热量传递的被动式冷却技术成为备受关注的研究热点。辐射制冷技术可以反射太阳光并通过“大气窗口”将热量辐射到外太空使物体表面自发冷却,因其不消耗任何能源就可以实现辐射降温而受到了国内外研究者的广泛关注。近年来,辐射冷却技术在建筑领域的应用快速发展,面临的主要问题是制备工艺复杂、生产成本较高和易受外界环境影响等。本文从辐射冷却原理出发,对最近几年辐射冷却材料在建筑领域的相关研究进行了归纳和总结,重点介绍了辐射冷却材料的制备技术以及影响辐射冷却性能的主要因素,并进一步按照主动式建筑节能和被动式建筑节能分类,详细阐述了辐射冷却材料在建筑领域的应用情况,最后对其存在的问题以及未来的研究方向进行了总结和展望,指出未来的研究应着眼于探索工艺简单、可大批量制备的辐射冷却材料,开发低成本且多功能集成的应用产品,并建立具体的标准和法规。Abstract: Along with the large consumption of fossil energy, the human living environment is seriously threatened. Among them, the proportion of building energy consumption in the total energy consumption continues to rise, and the passive cooling technology that effectively manages the heat transfer of buildings has become a focus of attention. Radiative cooling technology can reflect sunlight and radiate heat to outer space through the "atmospheric window" to spontaneously cool the surface of objects. Because it can realize radiative cooling without consuming any energy, it has been widely concerned by researchers at home and abroad. In recent years, the application of radiative cooling technology in the field of construction has developed rapidly. The main problems are complex preparation process, high production cost and easy to be affected by external environment. Based on the principle of radiative cooling, this paper summarizes the relevant researches of radiative cooling materials in buildings in recent years, focuses on the preparation process of radiative cooling materials and the main factors affecting the performance of radiative cooling materials, and further elaborates the application of radiative cooling materials in buildings according to the classification of energy saving of active buildings and energy saving of passive buildings. Finally, the existing problems and the future research direction are summarized and prospected. It is pointed out that future research should focus on exploring the simple process of radiation cooling materials that can be prepared in large quantities, developing low-cost and multi-functional integrated application products, and establishing specific standards and regulations.
-
Key words:
- radiative cooling /
- building energy saving /
- passive cooling /
- porous polymer /
- solar energy
-
图 2 (a)PVDF的SEM图像和PVDF、TiO2-PVDF的红外光谱曲线[5];PDMS的SEM图像和吸收率[6];PMMA的SEM图像和反射率[29];(b)PTFE涂层纸复合材料的表面润湿状态、微观结构和光谱表征图[11]
Figure 2. (a) SEM images of PVDF and infrared spectral curves of PVDF、TiO2-PVDF [5]; SEM images and absorptivity of PDMS[6]; The SEM images and reflectance of PMMA[29] and the surface wetting state; (b) surface-wetting states and microstructures of PTFE-coated paper composites[11].
图 4 随机分布的粒子结构:(a)SiO2微球随机分布在TPX上以银为背衬的超材料结构示意图[14];(b)人体皮肤自然褶皱仿生结构涂层[25];(c)具有随机分布的MgO/PVDF纳米颗粒辐射冷却涂料[26]
Figure 4. Randomly distributed particle structure:(a) The schematic diagram of the metamaterial structure of SiO2 microspheres randomly distributed on TPX with silver as the substrate[14]; (b) Human skin natural fold bionic structure coating[25]; (c) Radiative cooling coatings with randomly distributed MgO / PVDF nanoparticles[26]
图 8 (a) 辐射冷却收集(RadiCold)模块示意图[54];(b)光伏结合辐射冷却系统(PV-RSC)[56];(c)新型辐射冷却辅助热电(PSC-TEC)系统[57]
Figure 8. (a) The schematic diagram of the radiation cooling collection (RadiCold) module[54];(b) Photovoltaic combined with radiation cooling system ( PV-RSC ) [56];(c) Radiation cooling assisted thermoelectric ( PSC-TEC ) system[57]
图 9 辐射冷却材料在建筑领域的应用:(a)原理示意图[68];(b)外墙及屋顶涂料[44];(c)墙体[61];(d)智能窗户[63];(e)冷却木材[64];(f)冷却纤维素板材和纯木板材的SEM图像[65]
Figure 9. Application of radiative cooling materials in architrcture: (a) Principle diagram[68];(b) The coating of exterior wall and roof[44];(c) Wall[61]; (d) Intelligent window[63];(e) Cooling wood[64];(f) The SEM images of the cooling lignocellulosic bulk and the pure wood fiber bulk[65]
-
[1] YU X, CHEN C. Coupling spectral-dependent radiative cooling with building energy simulation[J]. Building and Environment, 2021, 197: 107841. doi: 10.1016/j.buildenv.2021.107841 [2] GENTLE A R, SMITH G B. A Subambient Open Roof Surface under the Mid-Summer Sun[J]. Advanced Science, 2015, 2(9): 1500119. doi: 10.1002/advs.201500119 [3] ZHANG Q, WANG S, WANG X, et al. Recent Progress in Daytime Radiative Cooling: Advanced Material Designs and Applications[J]. Small Methods, 2022, 6(4): 2101379. doi: 10.1002/smtd.202101379 [4] LIN K-T, HAN J, LI K, et al. Radiat-ive cooling: Fundamental physics, atmospheric influences, materials and str-uctural engineering, applications and beyond[J]. Nano Energy, 2021, 80: 105517. doi: 10.1016/j.nanoen.2020.105517 [5] LI N, WEI L, YOU M, et al. Hierarchically structural TiO2-PVDF fiber film with particle-enhanced spectral performance for radiative sky cooling[J]. Solar Energy, 2023, 259: 41-48. doi: 10.1016/j.solener.2023.05.011 [6] WANG Y, WANG T, LIANG J, et al. Controllable-morphology polymer blend photonic metafoam for radiative cooling[J]. Materials Horizons, 2023, 10(11): 5060-5070. doi: 10.1039/D3MH01008B [7] TIAN Q, TU X, YANG L, et al. Super-Large-Scale Hierarchically Porous Films Based on Self-Assembled Eye-Like Air Pores for High-Performance Daytime Radiative Cooling[J]. Small, 2022, 18(51): 2205091. doi: 10.1002/smll.202205091 [8] LIU J, MA W, DENG X, et al. Superhydrophobic nanoparticle mixture coat-ing for highly efficient all-day radiati-ve cooling[J]. Applied Thermal Engi-neering, 2023, 228: 120490. doi: 10.1016/j.applthermaleng.2023.120490 [9] FENG J, ZHAI Q, ZHU Q. Research on improving heat dissipation of mon-ocrystalline silicon solar cells based o-n radiation cooling[J]. International J-ournal of Energy Research, 2022, 46(9): 12160-12172. doi: 10.1002/er.7981 [10] FENG J, GAO K, JIANG Y, et al. O-ptimization of random silica-polymethylpentene (TPX) radiative coolers tow-ards substantial cooling capacity[J]. S-olar Energy Materials and Solar Cells, 2022, 234: 111419. doi: 10.1016/j.solmat.2021.111419 [11] TIAN Y, SHAO H, LIU X, et al. Su-perhydrophobic and Recyclable Cellulose-Fiber-Based Composites for High-Ef-ficiency Passive Radiative Cooling[J]. ACS Applied Materials & Interfaces, 2021, 13(19): 22521-22530. [12] CHEN Z, ZHU L, RAMAN A, et al. Radiative cooling to deep sub-freezing temperatures through a 24-h day–night cycle[J]. Nature Communications, 2016, 7(1): 13729. doi: 10.1038/ncomms13729 [13] CHAE D, KIM M, JUNG P-H, et al. Spectrally Selective Inorganic-Based Multilayer Emitter for Daytime Radiative Cooling[J]. ACS Applied Materials & Interfaces, 2020, 12(7): 8073-8081. [14] ZHAI Y, MA Y, DAVID S N, et al. Scalable-manufactured randomized glass-polymer hybrid metamaterial for daytime radiative cooling[J]. Science, 2017, 355(6329): 1062-1066. doi: 10.1126/science.aai7899 [15] CHEN C, JIA X, LI X, et al. Scalable wet-spinning of wearable chitosan-silica textile for all-day radiative cooling[J]. Chemical Engineering Journal, 2023, 475: 146307. doi: 10.1016/j.cej.2023.146307 [16] FAN W, GAO Q, XIANG J, et al. Synergistic effect of silica aerogel and titanium dioxide in porous polyurethane composite coating with enhanced passive radiative cooling performance[J]. Progress in Organic Coatings, 2023, 183: 107763. doi: 10.1016/j.porgcoat.2023.107763 [17] LEE D, GO M, SON S, et al. Sub-ambient daytime radiative cooling by silica-coated porous anodic aluminum oxide[J]. Nano Energy, 2021, 79: 105426. doi: 10.1016/j.nanoen.2020.105426 [18] MA H, YAO K, DOU S, et al. Multilayered SiO2/Si3N4 photonic emitter to achieve high-performance all-day radiative cooling[J]. Solar Energy Materials and Solar Cells, 2020, 212: 110584. doi: 10.1016/j.solmat.2020.110584 [19] XIANG B, ZHANG R, LUO Y, et al. 3D porous polymer film with designed pore architecture and auto-deposited SiO2 for highly efficient passive radiative cooling[J]. Nano Energy, 2021, 81: 105600. doi: 10.1016/j.nanoen.2020.105600 [20] CHEN X, HE M, FENG S, et al. Cellulose-based porous polymer film with auto-deposited TiO2 as spectrally selective materials for passive daytime radiative cooling[J]. Optical Materials, 2021, 120: 111431. doi: 10.1016/j.optmat.2021.111431 [21] CHEN Y, MANDAL J, LI W, et al. Colored and paintable bilayer coatings with high solar-infrared reflectance for efficient cooling[J]. Science Advances, 2020, 6(17): eaaz5413. doi: 10.1126/sciadv.aaz5413 [22] SUN L, LIU Z, HUANG H, et al. Fl-exible Al2O3/polymethyl methacrylate composite nanofibers for high-perform-ance sun shading and radiative coolin-g[J]. Materials Today Communication-s, 2023, 37: 106903. doi: 10.1016/j.mtcomm.2023.106903 [23] RAMAN A P, ANOMA M A, ZHU L, et al. Passive radiative cooling below ambient air temperature under direct sunlight[J]. Nature, 2014, 515(7528): 540-544. doi: 10.1038/nature13883 [24] MABCHOUR G, BENLATTAR M, SAADOUNI K, et al. Daytime radiative cooling purposes with selective multilayer design based on Ta2O5[J]. Optik, 2020, 214: 164811. doi: 10.1016/j.ijleo.2020.164811 [25] CHENG Z, HAN H, WANG F, et al. Efficient radiative cooling coating with biomimetic human skin wrinkle structure[J]. Nano Energy, 2021, 89: 106377. doi: 10.1016/j.nanoen.2021.106377 [26] DAS P, RUDRA S, MAURYA K C, et al. Ultra-Emissive MgO-PVDF Polymer Nanocomposite Paint for Passive Daytime Radiative Cooling[J]. Advanced Materials Technologies, 2023, 8(24): 2301174. doi: 10.1002/admt.202301174 [27] HUANG J, FAN D, LI Q. Structural rod-like particles for highly efficient radiative cooling[J]. Materials Today Energy, 2022, 25: 100955. doi: 10.1016/j.mtener.2022.100955 [28] CAI H, FENG S, FENG M, et al. Co-ral-Inspired Asymmetrically Porous R-adiative Cooling Biofilm with Thermo-plastic Polyurethane-Enhanced Mechan-ical Tolerance as Building Energy-Sav-ing Envelope[J]. ACS Applied Poly-mer Materials, 2023, 5(12): 10053-10064. doi: 10.1021/acsapm.3c01914 [29] WANG T, WU Y, SHI L, et al. A structural polymer for highly efficient all-day passive radiative cooling[J]. Nature Communications, 2021, 12(1): 365. doi: 10.1038/s41467-020-20646-7 [30] MISHRA R K, MISHRA P, VERMA K, et al. Electrospinning production of nanofibrous membranes[J]. Environmental Chemistry Letters, 2018, 17(2): 767-800. [31] KONG L, SUN P, LIU J, et al. Superhydrophobic and mechanical properties enhanced the electrospinning film with a multiscale micro-nano structure for high-efficiency radiation cooling[J]. Journal of Materials Chemistry A, 2024, 12(13): 7886-7895. doi: 10.1039/D3TA07191J [32] JEON H, NA J H, KIM Y S, et al. Electrospinning-assisted radiative cooli-ng composite films[J]. Solar Energy Materials and Solar Cells, 2023, 255. [33] XIANG B, XU P, CHANG Y, et al. Biodegradable radiative cooling membrane based on electrospun silk fibroin fiber[J]. Polymers for Advanced Technologies, 2023, 34(5): 1716-1722. doi: 10.1002/pat.6005 [34] LI X, XU H, YANG Y, et al. Selective spectral absorption of nanofibers for color-preserving daytime radiative cooling[J]. Materials Horizons, 2023, 10(7): 2487-95. doi: 10.1039/D3MH00391D [35] TSAI M-T, CHANG S-W, CHEN Y-J, et al. Scalable, flame-resistant, superhydrophobic ceramic metafibers for sustainable all-day radiative cooling[J]. Nano Today, 2023, 48. 101745. [36] LI S, DU G, PAN M, et al. Scalable and sustainable hierarchical-morphology coatings for passive daytime radiative cooling[J]. Advanced Composites and Hybrid Materials, 2024, 7(1). [37] ZHANG W, WANG Y, SUN H, et al. Thermal conductive high-density polyethylene/boron nitride composites with high solar reflectivity for radiative cooling[J]. Advanced Composites and Hybrid Materials, 2023, 6(5): 163. doi: 10.1007/s42114-023-00739-9 [38] GONG Q, LU L, CHEN J, et al. A novel aqueous scalable eco-friendly paint for passive daytime radiative cooling in sub-tropical climates[J]. Solar Energy, 2023, 255: 236-242. doi: 10.1016/j.solener.2023.03.033 [39] JIANG T, FAN W, WANG F. Long-lasting self-cleaning daytime radiative cooling paint for building[J]. Colloids and Surfaces A: Physicochemical and Engineering Aspects, 2023, 666: 131296. doi: 10.1016/j.colsurfa.2023.131296 [40] CAI X, WANG Y, LUO Y, et al. Rationally Tuning Phase Separation in Polymeric Membranes toward Optimized All-day Passive Radiative Coolers[J]. ACS Applied Materials & Interfaces, 2022, 14(23): 27222-27232. [41] LI B, CAO B, SONG R, et al. Low-cost and scalable sub-ambient radiative cooling porous films[J]. Journal of Photonics for Energy, 2023, 13(1): 015501. [42] MANDAL J, FU Y, OVERVIG A C, et al. Hierarchically porous polymer coatings for highly efficient passive daytime radiative cooling[J]. Science, 2018, 362(6412): 315-319. doi: 10.1126/science.aat9513 [43] LI L, LIU G, ZHANG Q, et al. Porous Structure of Polymer Films Optimized by Rationally Tuning Phase Separation for Passive All-Day Radiative Cooling[J]. ACS Applied Materials & Interfaces, 2024, 16(5): 6504-6512. [44] WANG J, SUN J, GUO T, et al. High-Strength Flexible Membrane with Rational Pore Architecture as a Selective Radiator for High-Efficiency Daytime Radiative Cooling[J]. Advanced Materials Technologies, 2021, 7(1): 2100528. [45] LIU X, ZHANG M, HOU Y, et al. Hierarchically Superhydrophobic Stereo-Complex Poly (Lactic Acid) Aerogel for Daytime Radiative Cooling[J]. Advanced Functional Materials, 2022, 32(46): 2207414. doi: 10.1002/adfm.202207414 [46] MANDAL J, FU Y, OVERVIG A C, et al. Hierarchically porous polymer coatings for highly efficient passive daytime radiative cooling[J]. Science, 2018, 362(6412): 315-319. doi: 10.1126/science.aat9513 [47] ZHANG Y, WANG T, MEI X, et al. Ordered Porous Polymer Films for Highly Efficient Passive Daytime Radiative Cooling[J]. ACS Photonics, 2023, 10(9): 3124-3132. doi: 10.1021/acsphotonics.3c00494 [48] ZHAO X, LI T, XIE H, et al. A solution-processed radiative cooling glass[J]. Science, 2023, 382(6671): 684-691. doi: 10.1126/science.adi2224 [49] ZHANG J, YANG X, XU R, et al. An environmentally friendly porous PDMS film via a template method based for passive daytime radiative cooling[J]. Materials Letters, 2024, 357: 135686. doi: 10.1016/j.matlet.2023.135686 [50] WU W, LIN S, WEI M, et al. Flexible passive radiative cooling inspired by Saharan silver ants[J]. Solar Energy Materials and Solar Cells, 2020, 210: 110512. doi: 10.1016/j.solmat.2020.110512 [51] WANG T, XIAO Y, KING J L, et al. Bioinspired Switchable Passive Daytime Radiative Cooling Coatings[J]. ACS Applied Materials & Interfaces, 2023, 15(41): 48716-48724. [52] LIU S, SUI C, HARBINSON M, et al. A Scalable Microstructure Photonic Coating Fabricated by Roll-to-Roll “Defects” for Daytime Subambient Passive Radiative Cooling[J]. Nano Letters, 2023, 23(17): 7767-7774. doi: 10.1021/acs.nanolett.3c00111 [53] JEON S K, KIM J T, KIM M S, et al. Scalable, Patternable Glass-Infiltrated Ceramic Radiative Coolers for Energy-Saving Architectural Applications[J]. Advanced Science, 2023, 10(27). [54] ZHAO D, AILI A, ZHAI Y, et al. Subambient Cooling of Water: Toward Real-World Applications of Daytime Radiative Cooling[J]. Joule, 2019, 3(1): 111-123. doi: 10.1016/j.joule.2018.10.006 [55] WANG W, FERNANDEZ N, KATIPAMULA S, et al. Performance assessment of a photonic radiative cooling system for office buildings[J]. Renewable Energy, 2018, 118: 265-277. doi: 10.1016/j.renene.2017.10.062 [56] ZHANG C, CHEN L, ZHOU Z, et al. Cooling performance of all-orientated building facades integrated with photovoltaic-sky radiative cooling system in summer[J]. Renewable Energy, 2023, 217: 119193. doi: 10.1016/j.renene.2023.119193 [57] ZHAO D, YIN X, XU J, et al. Radiative sky cooling-assisted thermoelectric cooling system for building applications[J]. Energy, 2020, 190: 116322. doi: 10.1016/j.energy.2019.116322 [58] DONG Y, ZOU Y, LI X, et al. Introducing masking layer for daytime radiative cooling coating to realize high optical performance, thin thickness, and excellent durability in long-term outdoor application[J]. Applied Energy, 2023, 344: 121273. doi: 10.1016/j.apenergy.2023.121273 [59] WU Q, CUI Y, XIA G, et al. Passive daytime radiative cooling coatings with renewable self-cleaning functions[J]. Chinese Chemical Letters, 2024, 35(2): 108687. doi: 10.1016/j.cclet.2023.108687 [60] ZHONG H, LI Y, ZHANG P, et al. Hierarchically Hollow Microfibers as a Scalable and Effective Thermal Insulating Cooler for Buildings[J]. ACS Nano, 2021, 15(6): 10076-10083. doi: 10.1021/acsnano.1c01814 [61] SHEN D, YU C, WANG W. Investigation on the thermal performance of the novel phase change materials wall with radiative cooling[J]. Applied Thermal Engineering, 2020, 176: 115479. doi: 10.1016/j.applthermaleng.2020.115479 [62] TANG Y, TAO Q, CHEN Y, et al. Building envelopes with radiative cooling materials: A model for indoor thermal environment assessment based on climate adaptation[J]. Journal of Building Engineering, 2023, 74: 106869. doi: 10.1016/j.jobe.2023.106869 [63] WANG S, ZHOU Y, JIANG T, et al. Thermochromic smart windows with highly regulated radiative cooling and solar transmission[J]. Nano Energy, 2021, 89: 106440. doi: 10.1016/j.nanoen.2021.106440 [64] LI T, ZHAI Y, HE S, et al. A radiative cooling structural material[J]. Science, 2019, 364(6442): 760-3. doi: 10.1126/science.aau9101 [65] CHEN Y, DANG B, FU J, et al. Cellulose-Based Hybrid Structural Material for Radiative Cooling[J]. Nano Letters, 2020, 21(1): 397-404. [66] NIE X, YOO Y, HEWAKURUPPU H, et al. Cool White Polymer Coatings based on Glass Bubbles for Buildings[J]. Scientific Reports, 2020, 10(1): 6661. doi: 10.1038/s41598-020-63027-2 [67] ZHU Y, LUO H, YANG C, et al. Color-preserving passive radiative cooling for an actively temperature-regulated enclosure[J]. Light: Science & Applications, 2022, 11(1) : 122. [68] QIN M, XIONG F, AFTAB W, et al. Phase-change materials reinforced intelligent paint for efficient daytime radiative cooling[J]. iScience, 2022, 25(7): 104584. doi: 10.1016/j.isci.2022.104584 -
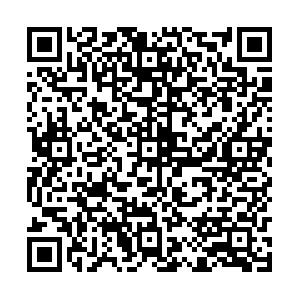
计量
- 文章访问数: 95
- HTML全文浏览量: 50
- 被引次数: 0