Stability and thermophysical characterization of ZrO2 nanoparticles by surface-modified
-
摘要: 纳米流体因具有优良的热物性被广泛用于换热设备中,但较差的稳定性限制了工业化应用。为了提高纳米流体的稳定性和传热性能,采用真空干燥法将β-环糊精(β-CD)接枝到ZrO2纳米颗粒表面,比对修饰前后纳米颗粒的形貌特征、表面官能团及分子结构变化情况。此外,采用两步法制备体积分数为0.06 vol.% 的ZrO2/乙二醇(EG):去离子水(DI)和β-ZrO2/EG:DI纳米流体,通过沉降观察法和透射电镜(TEM)共同表征修饰前后纳米流体的稳定程度,并研究了纳米流体在20-60 ℃温度下的黏度和导热系数的变化规律。实验结果表明,表面附着了聚合物的β-ZrO2纳米颗粒之间产生了空间位阻,削弱了颗粒的团聚趋势,有利于保持纳米流体的长期稳定。与室温下的ZrO2纳米流体相比,β-ZrO2纳米流体中颗粒分散更加均匀,静置2天后团聚体的沉降速度减小了57.90%。在60 ℃时,β-ZrO2/EG:DI的黏度与ZrO2/EG:DI纳米流体相比无明显变化,而导热系数增大了10.25%。这是因为,包裹在β-ZrO2纳米颗粒表面的聚合物形成了弹性层,使得已修饰颗粒间产生弹性碰撞,从而引起微对流达到强化换热的效果。因此,在不影响纳米流体热物性的前提下,纳米颗粒表面修饰是改善纳米流体稳定性的有效方式之一。Abstract: Nanofluids have attracted considerable interest in advanced thermal properties; however, poor stability limits practical application. Then, vacuum drying methods were used to graft β-cyclodextrin (β-CD) onto ZrO2 nanoparticles to improve stability of nanofluids. The morphological features, surface functional groups, and molecular structure of the nanoparticles before and after modification were compared. A two-step method was used to prepare ZrO2/EG:DI and β-ZrO2/EG:DI nanofluids at volume fraction of 0.06 vol.%. The stability of the nanofluids were characterized by TEM and sedimentation observation method, and the viscosity and thermal conductivity were evaluated in the temperature range of 20–60 ℃. The results indicated that when β-ZrO2 nanoparticles are modified with β-CD, the polymer attachment introduces spatial site resistance between the nanoparticles. This resistance mitigates particle agglomeration and promotes the long-term stability of the nanofluid. Therefore, the modified β-ZrO2 nanoparticles were more uniformly dispersed, which had a 57.90% lower settling velocity in base fluid than ZrO2 nanofluid at room temperature. Besides, the thermal conductivity of β-ZrO2 nanofluid was10.25% higher than that of ZrO2 nanofluid at 60 ℃ with the constant viscosity. The enhancement in nanofluid thermal conductivity results from the polymer wrapped around the β-ZrO2 nanoparticles, forming an elastic layer and leading to elastic collisions, as well as micro-convection. Therefore, surface modification of nanoparticles is one of the effective methods to improve the stability of nanofluids without affecting the thermal conductivity.
-
Key words:
- nanoparticles surface modification /
- β-cyclodextrin /
- nanofluids /
- stability /
- thermal conductivity
-
图 8 改性纳米颗粒稳定性及热物性增强机理 (a)弹性层分子结构;(b)空间位阻;(c) SEM;(d)沉降图;(e)弹性碰撞;(f)导热系数示意图
Figure 8. Mechanism of enhanced stability and thermophysical property of modified nanofluids: (a) molecular structure of the elastic layer; (b) steric potential resistance; (c) SEM; (d) sedimentation diagram; (e) elastic collision; (f) diagram of thermal conductivity.
表 1 纳米颗粒及基液的物理参数
Table 1. Physical Parameters of Nanoparticles and Base fluids
Material Density/
(kg·m−3)Specific heat capacity/
(J·kg−1·K−1)Thermal conductivity/
(W·m−1·K−1)Manufacturers ZrO2 nanoparticles 5850 713 2 Beijing Deke Daojin Science and Technology Ethylene glycol 1110 2395 0.256 Shanghai Aladdin Biochemical Technology Deionized water 998.2 4185 0.599 Labs -
[1] 张涛, 周园园, 贾楠楠. 钢铁行业余热资源分布与特点[J]. 冶金动力, 2023, (5): 108-113.ZHANG Tao, ZHOU Yuanyuan, JIA Nannan. Distribution and Characteristics of Waste Heat Resources in Iron and Steel Industry.[J]. Metallurgical Power, 2023, (5): 108-113(in Chinese). [2] ONYIRIUKA E. Modelling the thermal conductivity of nanofluids using a novel model of models approach[J]. Journal of Thermal Analysis and Calorimetry, 2023, 148(23): 13569-13585. doi: 10.1007/s10973-023-12642-y [3] CHEN W, ZHAI Y, BAI Y, et al. Heat transfer mechanism for abnormal enhancement of thermal conductivity in a nanofluidic system by molecular dynamics[J]. Powder Technology, 2024, 433: 119132. doi: 10.1016/j.powtec.2023.119132 [4] GARCIA-RINCÓN M A, FLORES-PRIETO J J. Nanofluids stability in flat-plate solar collectors: A review[J]. Solar Energy Materials and Solar Cells, 2024, 271: 112832. doi: 10.1016/j.solmat.2024.112832 [5] LI Y, ZHAI Y, CHEN W, et al. Microscopic mechanisms of particle agglomeration to enhance transport properties of nanofluids[J]. Journal of Molecular Liquids, 2023, 383: 122008. doi: 10.1016/j.molliq.2023.122008 [6] GUO W, ZHAI Y. Optimizing stability and enhancing flow boiling heat transfer performance of Al2O3-TiO2/water hybrid nanofluids. Experimental Thermal and Fluid Science, 2024: 111177. [7] 熊亚选, 宋超宇, 药晨华, 等. 纳米流体稳定性研究综述[J]. 华电技术, 2021, 43(7): 68-74.XIONG Yaxuan, SONG Chaoyu, YAO Chenhua, et al. Review on the stability of nanofluids[J]. Huadian Technology, 2021, 43(7): 68-74(in Chinese). [8] KAGGWA A, ATKINS M, TARIGHALESLAMI A, et al. Thermal Performance of Selected Nanofluids with Surfactants[J]. International Journal of Thermophysics, 2023, 44(11). [9] CHAKRABORTY S, SARKAR I, BEHERA D K, PAL S K. Experimental investigation on the effect of dispersant addition on thermal and rheological characteristics of TiO2 nanofluid[J]. Powder Technology, 2017, 307: 10-24. doi: 10.1016/j.powtec.2016.11.016 [10] BAEK S, SHIN D, KIM G, et al. Influence of amphoteric and anionic surfactants on stability, surface tension, and thermal conductivity of Al2O3/water nanofluids[J]. Case Studies in Thermal Engineering, 2021, 25: 100995. doi: 10.1016/j.csite.2021.100995 [11] SEN S, GOVINDARAJAN V, PELLICCIONE C J, et al. Surface Modification Approach to TiO2 Nanofluids with High Particle Concentration, Low Viscosity, and Electrochemical Activity[J]. ACS Applied Materials & Interfaces, 2015, 7(37): 20538-20547. [12] OLIVEIRA L R, SILVA A C A, DANTAS N O, et al. Thermophysical properties of TiO2-PVA/water nanofluids[J]. International Journal of Heat and Mass Transfer, 2017, 115: 795-808. doi: 10.1016/j.ijheatmasstransfer.2017.07.094 [13] WANG H, ZOU C. β-Cyclodextrin modified TiO2 nanofluids to improve the stability and thermal conductivity of oil-based drilling fluids[J]. Colloids and Surfaces A: Physicochemical and Engineering Aspects, 2023, 661: 130957. doi: 10.1016/j.colsurfa.2023.130957 [14] HĂDARUGĂ N G, BANDUR G N, DAVID I, et al. A review on thermal analyses of cyclodextrins and cyclodextrin complexes[J]. Environmental chemistry letters, 2019, 17(1): 349-373. doi: 10.1007/s10311-018-0806-8 [15] WANG Y, ZOU C, LI W, et al. Improving stability and thermal properties of TiO2 nanofluids by supramolecular modification: high energy efficiency heat transfer medium for data center cooling system[J]. International Journal of Heat and Mass Transfer, 2020, 156: 119735. doi: 10.1016/j.ijheatmasstransfer.2020.119735 [16] KAMALGHARIBI M, HORMOZI F, ZAMZAMIAN S, et al. Experimental studies on the stability of CuO nanoparticles dispersed in different base fluids: influence of stirring, sonication and surface-active agents. [J] Heat and Mass Transfer, 2016;52(1): 55-62. [17] HARRISON R G, TODD P W, RUDGE S R, et al. [M] Bioseparations science and engineering. Oxford University Press; 2015. [18] ZHAI Y, XIA G, LIU X, et al. Heat transfer enhancement of Al2O3-H2O nanofluids flowing through a micro heat sink with complex structure[J]. International Communication in Heat and Mass Transfer, 66 (2015): 158-166. [19] M. H. ESFE, H. ROSTAMIAN, M. R. SARLAK. A novel study on rheological behavior of ZnO-MWCNT/10w40 nanofluid for automotive engines[J]. Journal of Molecular Liquids, 254 (2018): 406-413. [20] 杨世铭, 陶文铨. 传热学[M]. 4版. 北京: 高等教育出版社, 2006: 563.YANG Shiming, TAO Wenquan. Heat transfer [M]. 4th ed. Beijing: Higher Education Press, 2006: 563 (in Chinese). [21] 马明琰. 混合纳米流体热物性及对流换热特性实验研究[D]. 昆明理工大学, 2021.MA Mingyan. Experimental study on thermophysical properties and convective heat transfer characteristics of hybrid nanofluids[D]. Kunming University of Science and Technology, 2021(in Chinese). [22] S. HALEFADL, T. MARÉ, P. ESTELLÉ. Efficiency of carbon nanotubes water based nanofluids as coolants[J]. Experimental Thermal and Fluid Science, 53 (2014): 104-110. [23] S. K. SINGH, J. SARKAR. Energy, exergy and economic assessments of shell and tube condenser using hybrid nanofluid as coolant[J]. International Communication in Heat and Mass Transfer, 98 (2018): 41-48. [24] PENG B, LIAO P, JIANG Y. Meta-Analysis to Revisit the Property-Aggregation Relationships of Carbon Nanomaterials: Experimental Observations versus Predictions of the DLVO Theory[J]. Langmuir, 2024, 40(13): 7127-7138. doi: 10.1021/acs.langmuir.4c00274 [25] DERJAGUIN B, LANDAU L. Theory of the stability of strongly charged lyophobic sols and of the adhesion of strongly charged particles in solutions of electrolytes. [J] Progress in Surface Science, 1993; 43: 30-59. -
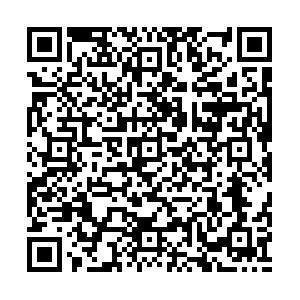
计量
- 文章访问数: 19
- HTML全文浏览量: 22
- 被引次数: 0