Enhancement Strategies of Output Performance of Triboelectric Nanogenerator
-
摘要: 摩擦纳米发电机(Triboelectric nanogenerator,TENG)是一类能将机械能转换为电能的电子设备,具有材料种类丰富、器件结构简单以及易于集成等特点,在蓝色能源收集、微/纳能源、自驱动传感等方面展示出广泛的应用前景。然而,如何提高TENG的输出性能一直是科学界关注的焦点。基于此,本文在查阅大量文献的基础上,从TENG的工作原理出发,分析了摩擦电材料、摩擦层结构和器件结构对TENG输出性能的影响,并总结了提升TENG输出性能的有效策略,最后对TENG今后的发展趋势进行了展望。Abstract: Triboelectric nanogenerator (TENG) is an electronic device, that exchanges mechanical energy into electrical energy. TENG has presented a wide range of potential applications, such as blue energy harvesting, micro/nano energy sources, and self-powered sensing, owing to abundant material, simple structures, and ease of integration. However, enhancing the output performance of TENG has remained a focal point of scientific interest. Hence, insulting many studies, this work has analyzed the influence of triboelectric materials, friction layer structures, and device structures on TENG output performance according to the working principle of TENG. Meanwhile, the enhancement strategies of TENG output performance are summarized. Finally, the future development of TENG is concluded.
-
Key words:
- Triboelectric nanogenerator /
- Friction layer /
- Device structure /
- Self-powered /
- Sensors
-
图 7 (a)离子注入聚四氟乙烯形成TENG的示意图[58];(b)正电荷注入聚酰亚胺形成TENG的示意图[59];(c)浮动层结构与电荷泵结合的TENG示意图[60]
Figure 7. (a) Schematic diagram of ion injection to form TENG[58];(b) schematic diagram of positive charge injection into polyimide to form TENG[59];(c) Schematic diagram of TENG with floating layer structure bound to the charge pump[60]
图 8 (a)改性丙烯酸树脂涂层作为电荷掠夺层的液-固TENG示意图[65];(b)含有聚(偏氟乙烯)-六氟丙烯共聚物和钛酸钡纳米颗粒的介电调制多孔复合涂层作为电荷掠夺层的织物TENG示意图[66]
Figure 8. (a) Schematic diagram of liquid-solid TENG of modified acrylic resin coating as charge plunder layer [65]; (b) schematic diagram of dielectric modulated porous composite coating containing poly (vinylidene fluoride) -hexafluoropropylene copolymer and barium titanate nanoparticles as charge plunder layer [66]
表 1 提升摩擦纳米发电机输出性能不同策略的优缺点
Table 1. Advantages and disadvantages of different strategies to improve the output performance of friction nanogenerators
策略 方法 优点 缺点 参考
文献摩擦电材料
加工材料复合 有机-有机复合 有机材料的复合不易出现团聚现象,所制备的TENG具有良好的稳定性 相较无机材料,有机材料的介电常数通常较小,有机材料复合对TENG输出性能提升有限 [28]
[29]
有机-无机复合有机-无机复合可有效调控材料的介电常数,并且可增加材料表面粗糙度,增大正负摩擦层间的有效接触面积 无机材料与有机材料的复合过程中存在无机材料分布不均、团聚等问题,导致表面电荷分布不均匀,影响TENG的输出稳定性 [30]
[31]材料表面
改性官能团引入 官能团的引入直接增强摩擦层的电荷转移能力,且不会破坏材料本身特性 材料表面出现磨损,官能团引入提高的TENG输出性能效益就会降低 [44]
[45]构建表面微纳结构 表面微纳结构可增大摩擦层间的有效接触面积,提高表面电荷密度 微纳结构的精度难以控制,且微纳结构易变形受损,影响TENG输出稳定性 [53]
[54]电荷注入 直接将外加电荷作用于材料表面,显著提高电荷密度 注入的电荷易耗散,影响TENG输出稳定性和耐久性,且技术要求高、成本高 [58]
[59]
[60]摩擦层结构
设计摩擦层功能分区 电荷掠夺层 直接增强摩擦层的电荷掠夺能力,显著增大材料间的电荷转移 功能层如何优化的相关理论研究还需进一步明晰 [64]
[65]电荷传输层 提高电荷迁移率,减小表面电荷消散,有利电荷存储 [66]
[67]电荷存储层 增强材料电荷存储能力,显著提高表面电荷密度 [68]
[69]器件结构
设计接触分离模式 输出电压高,对压力变化感应敏感 对正负摩擦层贴合度有一定要求 [72]
[73]横向滑动模式 能量转化率高,适用于检测平面运动 摩擦层材料易受损 [74]
[75]单电极模式 易与其他设备进行集成,相较其他类型具有更广阔的应用前景 输出性能较其他模式低 [76]
[77]独立摩擦模式 摩擦层可移动,适用于检测移动物体的运动 摩擦层材料易受损,且对运动频率有一定要求 [78]
[79] -
[1] 肖先勇, 郑子萱. “双碳” 目标下新能源为主体的新型电力系统: 贡献, 关键技术与挑战[J]. 工程科学与技术, 2022, 54(1): 47-59.XU Xianyong, ZHENG Zixuan. New Power Systems Dominated by Renewable Energy Towards the Goal of Emission Peak & Carbon Neutrality: Contribution, Key Techniques, and Challenges[J]. Advanced Engineering Science, 2022, 54(1): 47-59 (in Chinese). [2] 于贵瑞, 郝天象, 朱剑兴. 中国碳达峰, 碳中和行动方略之探讨[J]. 中国科学院院刊, 2022, 37(4): 423-434.YU Guirui, HAO Tianxiang, ZHU Jianxing. Discussion on action strategies of China’s carbon peak and carbon neutrality[J]. Bulletin of Chinese Academy of Sciences, 2022, 37(4): 423-434 (in Chinese). [3] FAN F R, TIAN Z Q, WANG Z L. Flexible triboelectric generator[J]. Nano energy, 2012, 1(2): 328-334. doi: 10.1016/j.nanoen.2012.01.004 [4] CHEN K, LI Y, YANG G, et al. Fabric-Based TENG Woven with Bio-Fabricated Superhydrophobic Bacterial Cellulose Fiber for Energy Harvesting and Motion Detection[J]. Advanced Functional Materials, 2023: 2304809. [5] HAO D, GONG Y, WU J, et al. A Self-Sensing and Self-Powered Wearable System Based on Multi-Source Human Motion Energy Harvesting[J]. Small, 2024: 2311036. [6] SHI B, WANG Q, SU H, et al. Progress in recent research on the design and use of triboelectric nanogenerators for harvesting wind energy[J]. Nano Energy, 2023: 108789. [7] SUN F, ZHU Y, JIA C, et al. Advances in self-powered sports monitoring sensors based on triboelectric nanogenerators[J]. Journal of Energy Chemistry, 2023, 79: 477-488. doi: 10.1016/j.jechem.2022.12.024 [8] 谭杜娟. 用于海洋能收集的摩擦纳米发电机的结构设计及性能研究[D]. 重庆: 重庆大学, 2022.TAN Dujuan. Structural Design and Property Study of Triboelectric Nanogenerator for Ocean Energy Harvesting[D]. Chongqing: University Of Chongqing, 2022(in Chinese). [9] SHAN C, HE W, WU H, et al. Dual Mode TENG with Self-Voltage Multiplying Circuit for Blue Energy Harvesting and Water Wave Monitoring[J]. Advanced Functional Materials, 2023: 2305768. [10] FAN F R, TIAN Z Q, WANG Z L. Flexible triboelectric generator[J]. Nano energy, 2012, 1(2): 328-334. doi: 10.1016/j.nanoen.2012.01.004 [11] MAHAPATRA A, AJIMASHA R S, DEEPAK D, et al. Textile-integrated MoS2-PDMS single electrode triboelectric nanogenerator for vibrational energy harvesting and biomechanical motion sensing[J]. Nano Energy, 2023, 116: 108829. doi: 10.1016/j.nanoen.2023.108829 [12] ONG D T K, KOAY J S C, SIM M T, et al. High performance composition-tailored PVDF triboelectric nanogenerator enabled by low temperature-induced phase transition[J]. Nano Energy, 2023, 113: 108555. doi: 10.1016/j.nanoen.2023.108555 [13] LI C, WANG P, ZHANG D. Self-healable, stretchable triboelectric nanogenerators based on flexible polyimide for energy harvesting and self-powered sensors[J]. Nano Energy, 2023, 109: 108285. doi: 10.1016/j.nanoen.2023.108285 [14] HAN G H, LEE S H, GAO J, et al. Sustainable charged composites with amphiphobic surfaces for harsh environment–tolerant non-contact mode triboelectric nanogenerators[J]. Nano Energy, 2023, 112: 108428. doi: 10.1016/j.nanoen.2023.108428 [15] WANG Y F, CAO B, YANG Y W, et al. Multi-channel self-powered attitude sensor based on triboelectric nanogenerator and inertia[J]. Nano Energy, 2023, 107: 108164. doi: 10.1016/j.nanoen.2022.108164 [16] SUN X, DONG L, LIU Y, et al. Biomimetic PVA-PVDF-based triboelectric nanogenerator with MXene doping for self-powered water sterilization[J]. Materials Today Nano, 2023, 24: 100410. doi: 10.1016/j.mtnano.2023.100410 [17] GU L, GERMAN L, LI T, et al. Energy harvesting floor from commercial cellulosic materials for a self-powered wireless transmission sensor system[J]. ACS Applied Materials & Interfaces, 2021, 13(4): 5133-5141. [18] CHEN Z, ZHOU C, XIA W, et al. Strong, flexible and robust aramid nanofibers-based textile-triboelectric nanogenerators for high temperature escape monitoring and multifunctional applications[J]. Nano Energy, 2024: 109359. [19] WANG Z, WAN D, CUI X, et al. Wearable electronics powered by triboelectrification between hair and cloth for monitoring body motions[J]. Energy Technology, 2022, 10(6): 2200195. doi: 10.1002/ente.202200195 [20] MATIN N A, MOHSENIAN R, RAYEGANI A, et al. Skin-Contact Triboelectric Nanogenerator for Energy Harvesting and Motion Sensing: Principles, Challenges, and Perspectives[J]. Biosensors, 2023, 13(9): 872.002 [21] CHEN X, LIU Y, SUN Y, et al. Electron trapping & blocking effect enabled by MXene/TiO2 intermediate layer for charge regulation of triboelectric nanogenerators[J]. Nano Energy, 2022, 98: 107236. doi: 10.1016/j.nanoen.2022.107236 [22] LI W, XIANG Y, ZHANG W, et al. Ordered mesoporous SiO2 nanoparticles as charge storage sites for enhanced triboelectric nanogenerators[J]. Nano Energy, 2023, 113: 108539. doi: 10.1016/j.nanoen.2023.108539 [23] XI B, WANG L, YANG B, et al. Boosting output performance of triboelectric nanogenerator based on BaTiO3: La embedded nanofiber membrane for energy harvesting and wireless power transmission[J]. Nano Energy, 2023, 110: 108385.6. [24] LEE K, HAN H S, RYU J H, et al. Laser-driven formation of ZnSnO3/CNT heterostructure and its critical role in boosting performance of the triboelectric nanogenerator[J]. Carbon, 2023, 212: 118120. doi: 10.1016/j.carbon.2023.118120 [25] SHRESTHA K, SHARMA S, PRADHAN G B, et al. A Siloxene/Ecoflex Nanocomposite-Based Triboelectric Nanogenerator with Enhanced Charge Retention by MoS2/LIG for Self-Powered Touchless Sensor Applications[J]. Advanced Functional Materials, 2022, 32(27): 2113005. doi: 10.1002/adfm.202113005 [26] GIUSEPPINA P , ANTONIO E D R C , ALESSIO L, et al. 2D Materials-based Electrochemical Triboelectric Nanogenerators[J]. Advanced Materials, 2023: 2211037. [27] ZOU H, ZHANG Y, GUO L, et al. Quantifying the triboelectric series[J]. Nature communications, 2019, 10(1): 1427. doi: 10.1038/s41467-019-09461-x [28] CANDIDO I C M, OIVEIRAl G S, RIBEIRO S J L, et al. PVA-silk fibroin bio-based triboelectric nanogenerator[J]. Nano Energy, 2023, 105: 108035. doi: 10.1016/j.nanoen.2022.108035 [29] BAI Z, XU Y, ZHANG Z, et al. Highly flexible, porous electroactive biocomposite as attractive tribopositive material for advancing high-performance triboelectric nanogenerator[J]. Nano Energy, 2020, 75: 104884. doi: 10.1016/j.nanoen.2020.104884 [30] CHEON S, KANG H, KIM H, et al. High-performance triboelectric nanogenerators based on electrospun polyvinylidene fluoride–silver nanowire composite nanofibers[J]. Advanced Functional Materials, 2018, 28(2): 1703778. doi: 10.1002/adfm.201703778 [31] LI W, LU L, YAN F, et al. High-performance triboelectric nanogenerators based on TPU/mica nanofiber with enhanced tribo-positivity[J]. Nano Energy, 2023, 114: 108629. doi: 10.1016/j.nanoen.2023.108629 [32] XU J, ZOU Y, NASHALIAN A, et al. Leverage surface chemistry for high-performance triboelectric nanogenerators[J]. Frontiers in Chemistry, 2020, 8: 577327. doi: 10.3389/fchem.2020.577327 [33] MORADI F, KARIMZADEH F, KHARAZIHA M. Rational micro/nano-structuring for high-performance triboelectric nanogenerator[J]. Journal of Alloys and Compounds, 2023, 960: 170693. doi: 10.1016/j.jallcom.2023.170693 [34] WANG S, XIE Y, NIU S, et al. Maximum surface charge density for triboelectric nanogenerators achieved by ionized-air injection: methodology and theoretical understanding[J]. Advanced Materials, 2014, 26(39): 6720-6728. doi: 10.1002/adma.201402491 [35] WU C, WANG A C, DING W, et al. Triboelectric nanogenerator: a foundation of the energy for the new era[J]. Advanced Energy Materials, 2019, 9(1): 1802906. doi: 10.1002/aenm.201802906 [36] WANG Z L, WANG A C. On the origin of contact-electrification[J]. Materials Today, 2019, 30: 34-51. doi: 10.1016/j.mattod.2019.05.016 [37] PENG Z, SONG J, GAO Y, et al. A fluorinated polymer sponge with superhydrophobicity for high-performance biomechanical energy harvesting[J]. Nano Energy, 2021, 85: 106021. doi: 10.1016/j.nanoen.2021.106021 [38] SHI L, KALE V S, TIAN Z, et al. Fluorinated Covalent Organic Framework as a Positive Tribo-Material for High-Performance Triboelectric Nanogenerators[J]. Advanced Functional Materials, 2023, 33(12): 2212891. doi: 10.1002/adfm.202212891 [39] LING Z, LIN F, HUANG X, et al. Influence of Molecular Structure and Material Properties on the Output Performance of Liquid–Solid Triboelectric Nanogenerators[J]. Micromachines, 2023, 14(10): 1825. doi: 10.3390/mi14101825 [40] YAO C, YIN X, YU Y, et al. Chemically functionalized natural cellulose materials for effective triboelectric nanogenerator development[J]. Advanced Functional Materials, 2017, 27(30): 1700794. doi: 10.1002/adfm.201700794 [41] SHIN S H, KWON Y H, KIM Y H, et al. Triboelectric charging sequence induced by surface functionalization as a method to fabricate high performance triboelectric generators[J]. ACS nano, 2015, 9(4): 4621-4627. doi: 10.1021/acsnano.5b01340 [42] YUAN H, ZHANG J, RENCUS L S, et al. The engineering of molecular packing in amino acid crystals for the enhanced triboelectric effect[J]. Nano Energy, 2023, 110: 108375. doi: 10.1016/j.nanoen.2023.108375 [43] THAKUR D, SEO S, HYUN J. Three-dimensional triboelectric nanogenerator with carboxymethylated cellulose nanofiber and perfluoroalkoxy films[J]. Journal of Industrial and Engineering Chemistry, 2023, 123: 220-229. doi: 10.1016/j.jiec.2023.03.037 [44] WANG S, ZI Y, ZHOU Y S, et al. Molecular surface functionalization to enhance the power output of triboelectric nanogenerators[J]. Journal of Materials Chemistry A, 2016, 4(10): 3728-3734. doi: 10.1039/C5TA10239A [45] SHIN S H, KWON Y H, Kim Y H, et al. Triboelectric charging sequence induced by surface functionalization as a method to fabricate high performance triboelectric generators[J]. ACS nano, 2015, 9(4): 4621-4627. doi: 10.1021/acsnano.5b01340 [46] 周亮. 基于仿生结构及可重构材料的摩擦纳米发电机设计与传感应用研究 [D]. 吉林: 吉林大学, 2022.ZHOU Liang. Design and Sensing Applications of Triboelectric Nanogenerators Based on Biomimetic Structure and Reconfigurable Materials [D]. Jilin: Jilin University, 2022(in Chinese). [47] 岳欧阳. 基于生物质胶原的多功能柔性电子传感材料的构建及性能研究 [D]. 西安: 陕西科技大学, 2022.YUE O Y. Preparation and Exploration of Multifunctional Collagen-basedFlexible Electronic Sensing Materials [D]. Xi'an: Shaanxi University of Technology , 2022(in Chinese). [48] MUTHU M, PANDEY R, WANG X, et al. Enhancement of triboelectric nanogenerator output performance by laser 3D-Surface pattern method for energy harvesting application[J]. Nano Energy, 2020, 78: 105205. doi: 10.1016/j.nanoen.2020.105205 [49] SHANG W, GU G Q, YANG F, et al. A sliding-mode triboelectric nanogenerator with chemical group grated structure by shadow mask reactive ion etching[J]. ACS nano, 2017, 11(9): 8796-8803. doi: 10.1021/acsnano.7b02866 [50] ZHANG S, RANS S M S, BHATTA T, et al. 3D printed smart glove with pyramidal MXene/Ecoflex composite-based toroidal triboelectric nanogenerators for wearable human-machine interaction applications[J]. Nano Energy, 2023, 106: 108110. doi: 10.1016/j.nanoen.2022.108110 [51] LIU Z, ZHAO Z, ZENG X, et al. Expandable microsphere-based triboelectric nanogenerators as ultrasensitive pressure sensors for respiratory and pulse monitoring[J]. Nano Energy, 2019, 59: 295-301. doi: 10.1016/j.nanoen.2019.02.057 [52] PINMING C, WONGWIRIYAPAN W, RATTANAMAI S, et al. Carbon nanotube/polydimethylsiloxane composite micropillar arrays using non-lithographic silicon nanowires as a template for performance enhancement of triboelectric nanogenerators[J]. Nanotechnology, 2020, 32(9): 095303. [53] GAO C, TONG W, LIU S, et al. Fully degradable chitosan–based triboelectric nanogenerators applying in disposable medical products for information transfer[J]. Nano Energy, 2023: 108876. [54] CHEN H, XU Y, ZHANG J, et al. Enhanced stretchable graphene-based triboelectric nanogenerator via control of surface nanostructure[J]. Nano Energy, 2019, 58: 304-311. doi: 10.1016/j.nanoen.2019.01.029 [55] GAO M, KIM S B, LI Y, et al. Triboelectric nanogenerator with enhanced output and durability based on Si-DLC films[J]. Nano Energy, 2023, 105: 107997. doi: 10.1016/j.nanoen.2022.107997 [56] PARK S H, LEE J, KONG D S, et al. Laminating Structure for Interlayer Corona Discharge Treatment Toward Ion-Based Nanogenerators[J]. Small Methods, 2023: 2300097. [57] XIAO Z, LUO Y, YUAN H, et al. Coupling charge pump and BUCK circuits to efficiently enhance the output performance of triboelectric nanogenerator[J]. Nano Energy, 2023, 115: 108749. doi: 10.1016/j.nanoen.2023.108749 [58] FAN Y, LI S, TAO X, et al. Negative triboelectric polymers with ultrahigh charge density induced by ion implantation[J]. Nano Energy, 2021, 90: 106574. doi: 10.1016/j.nanoen.2021.106574 [59] WU H, FU S, HE W, et al. Improving and quantifying surface charge density via charge injection enabled by air breakdown[J]. Advanced Functional Materials, 2022, 32(35): 2203884. doi: 10.1002/adfm.202203884 [60] XU L, BU T Z, YANG X D, et al. Ultrahigh charge density realized by charge pumping at ambient conditions for triboelectric nanogenerators[J]. Nano Energy, 2018, 49: 625-633. doi: 10.1016/j.nanoen.2018.05.011 [61] SEIFOLLAHI Z, ASHRAFIZADEH S N. Ionic-size dependent electroosmotic flow in ion-selective biomimetic nanochannels[J]. Colloids and Surfaces B: Biointerfaces, 2022, 216: 112545. doi: 10.1016/j.colsurfb.2022.112545 [62] CHAI B, SHI K, ZOU H, et al. Conductive interlayer modulated ferroelectric nanocomposites for high performance triboelectric nanogenerator[J]. Nano Energy, 2022, 91: 106668. doi: 10.1016/j.nanoen.2021.106668 [63] JIANG H, LEI H, WEN Z, et al. Charge-trapping-blocking layer for enhanced triboelectric nanogenerators[J]. Nano Energy, 2020, 75: 105011. doi: 10.1016/j.nanoen.2020.105011 [64] KIM D W, LEE J H, YOU I, et al. Adding a stretchable deep-trap interlayer for high-performance stretchable triboelectric nanogenerators[J]. Nano Energy, 2018, 50: 192-200. doi: 10.1016/j.nanoen.2018.05.041 [65] WANG H, FENG M, WANG W, et al. Fluorine-free coating-based droplet triboelectric nanogenerators for highly efficient energy harvesting[J]. Journal of Materials Chemistry A, 2024, 12(8): 4727-4738. doi: 10.1039/D3TA07103K [66] ZHENG Z, MA X, LU M, et al. High-Performance All-Textile Triboelectric Nanogenerator toward Intelligent Sports Sensing and Biomechanical Energy Harvesting[J]. ACS Applied Materials & Interfaces, 2024, 16(8): 10746-10755. [67] YAN J, WANG H, WANG X, et al. High-performance triboelectric nanogenerators with laser-induced graphene pattern for efficient charge transfer[J]. Applied Surface Science, 2024, 661: 160034. doi: 10.1016/j.apsusc.2024.160034 [68] CUI N, LIU J, LEI Y, et al. High-performance triboelectric nanogenerator with a rationally designed friction layer structure[J]. ACS Applied Energy Materials, 2018, 1(6): 2891-2897. doi: 10.1021/acsaem.8b00530 [69] MENGE H G, KIM J O, PARK Y T. Enhanced triboelectric performance of modified PDMS nanocomposite multilayered nanogenerators[J]. Materials, 2020, 13(18): 4156. doi: 10.3390/ma13184156 [70] ZHENG Z, XIA J, WANG B, et al. Hierarchically designed nanocomposites for triboelectric nanogenerator toward biomechanical energy harvester and smart home system[J]. Nano Energy, 2022, 95: 107047. doi: 10.1016/j.nanoen.2022.107047 [71] LAI M, DU B, GUO H, et al. Enhancing the output charge density of TENG via building longitudinal paths of electrostatic charges in the contacting layers[J]. ACS applied materials & interfaces, 2018, 10(2): 2158-2165. [72] CUI N, LIU J, LEI Y, et al. High-performance triboelectric nanogenerator with a rationally designed friction layer structure[J]. ACS Applied Energy Materials, 2018, 1(6): 2891-2897. doi: 10.1021/acsaem.8b00530 [73] LIU D, YANG P, GAO Y, et al. A Dual-Mode Triboelectric Nanogenerator for Efficiently Harvesting Droplet Energy[J]. Small, 2024: 2400698. [74] LI Q, HU Y, YANG Q, et al. A robust constant–voltage DC triboelectric nanogenerator using the ternary dielectric triboelectrification effect[J]. Advanced Energy Materials, 2023, 13(2): 2202921. doi: 10.1002/aenm.202202921 [75] JEONG J, JEON S, MA X, et al. A Sustainable and Flexible Microbrush-Faced Triboelectric Generator for Portable/Wearable Applications[J]. Advanced Materials, 2021, 33(39): 2102530. doi: 10.1002/adma.202102530 [76] WU P, YANG P, LIU Z, et al. Boosting the Output of Liquid–Solid Triboelectric Nanogenerator by an External Charge-Pumping Strategy[J]. Advanced Energy Materials, 2024, 14(16): 2303912. doi: 10.1002/aenm.202303912 [77] HE M, DU W, FENG Y, et al. Flexible and stretchable triboelectric nanogenerator fabric for biomechanical energy harvesting and self-powered dual-mode human motion monitoring[J]. Nano Energy, 2021, 86: 106058. doi: 10.1016/j.nanoen.2021.106058 [78] PAOSANGTHONG W, WAGIH M, TORAH R, et al. Textile-based triboelectric nanogenerator with alternating positive and negative freestanding woven structure for harvesting sliding energy in all directions[J]. Nano Energy, 2022, 92: 106739. doi: 10.1016/j.nanoen.2021.106739 [79] SUN Y, YU Y, GAO Q, et al. Enhancing performance of triboelectric nanogenerator by accelerating the charge transfer strategy[J]. Nano Energy, 2024, 121: 109194. doi: 10.1016/j.nanoen.2023.109194 -
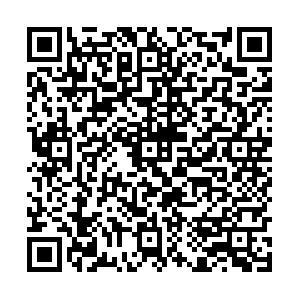
计量
- 文章访问数: 21
- HTML全文浏览量: 11
- 被引次数: 0