Research progress of polymer-based wave-absorbing and heat-conducting composites
-
摘要: 针对5G或6G通信设备、超级计算机、无线能量传输装置、AI智能、量子储存、VR技术和微波医疗器等精密电子设备朝着小型化和高度集成化发展所带来的电磁兼容和散热两大问题,研制兼具良好的绝缘性、缓震性、高效吸波性能以及优良导热能力的柔性吸波导热复合材料非常必要。本文从单一的电磁波吸收功能复合材料和散热性能复合材料的设计制备出发,归纳了电磁波吸波机理与导热机理以及影响吸波和导热性能的重要因素。在此基础上介绍了一些国内外聚合物基吸波导热复合材料的综合性能及其设计制备方法,在总结现有吸波导热多功能复合材料的研究现状和存在问题的基础上,考虑当前设计研发中存在的不足,提出了对于未来聚合物基吸波导热材料的发展方向的思考。此文旨在为制备高性能吸波导热复合材料材料提供思路,提升行业技术水平,开发出兼具高导热和优异电磁波吸收能力的新型复合材料。Abstract: Because of the two major problems of electromagnetic compatibility and heat dissipation brought about by the development of 5G or 6G communication devices, supercomputers, wireless energy transmission devices, AI intelligence, quantum storage, VR technology, and microwave medical devices and other precision electronic devices toward miniaturization and high integration, it is essential to develop flexible wave-absorbing and heat-conducting composites with both good insulating properties, vibration damping, high efficiency wave-absorbing properties, and excellent heat-conducting capabilities. In this paper, from the design and preparation of single electromagnetic wave absorbing composites and heat dissipating composites, the electromagnetic wave absorbing mechanism and thermal conductivity mechanism as well as the important factors affecting the wave absorbing and thermal conductivity performance are summarized. On this basis, the comprehensive performance of some domestic and foreign polymer-based wave-absorbing and heat-conducting composites and their design and preparation methods are introduced, and based on summarizing the current research status of existing wave-absorbing and heat-conducting multifunctional composites and the existing problems, and taking into consideration of the shortcomings in the current research and development of the current design, insights into the direction of the development of the polymer-based wave-absorbing and heat-conducting materials in the future are proposed. Through this study, we aim to provide a reference for the preparation of high-performance wave-absorbing and heat-conducting composite materials, improve the technical level of the industry, and develop new composite materials with both high thermal conductivity and excellent electromagnetic wave absorption ability.
-
图 5 (a) TiO2@C-Ni/CNTs制备过程示意图;(b) TiO2@C-Ni/CNTs的扫描电镜图片;(c) TiO2@C-Ni/CNTs的RL;(d) TiO2@C-Ni/CNTs的EAB;(f) 复合材料的热导率和热扩散系数[60]
Figure 5. (a) Schematic representation of the preparation process for TiO2@C-Ni/CNTs; (b) SEM images of TiO2@C-Ni/CNTs; (c) RL values and (d) EAB values of TiO2@C-Ni/CNTs; (f) The thermal diffusivity and thermal conductivity of composite [60]
图 9 (a) 垂直定向排列NiCO@CFs弹性体的制造示意图,以及CF、GO/CF和NiCO@CFs的扫描电镜图像;(b) 不同取向的NiCO@CFs弹性体的面外热导率;(c) 不同取向角和质量比NiCO@CFs弹性体的EAB和RLmin[66]
Figure 9. (a) Schematic illustration of fabrication for vertical orientation NiCO@CFs elastomer and the SEM images of CF, GO/CF, and NiCO@CFs, respectively; (b) The out-of-plane thermal conductivity of NiCO@CFs elastomers with different orientations; (c) EAB and RLmin of NiCO@CFs elastomer with different orientation angles and mass ratios [66]
Indicators Quantitative values RL/dB ≤ −10 EAB/GHz ≥2 λ (W·m−1·K−1) ≥ 1.5 volume resistivity (Ω·cm) ≥ 108 breakdown voltage (kV·mm−1) ≥ 1 ρ (g·cm−3) ≤ 3.5 shore hardness/HA ≤ 80 Thickness/mm 0.5-3 Notes: RL is the reflection loss; EAB is the effective absorption bandwidth; λ is the thermal conductivity;ρ is the density. 表 2 吸波导热复合材料性能比较
Table 2. Comparison of the performance of wave-absorption and heat-conduction composites
Materials Thickness/mm RL/dB EABmax/Ghz λ (W·m−1·K−1) References Al2O3/EG/EP 1.8 −43 2.8 0.76 [54] BaTiO3/EG/EP 1.6 −25.7 2.9 0.79 TiO2/EG/EP 1.4 −33.9 2.7 0.95 ZnO/EG/EP 1.8 −46 2.9 1.19 CIP/ZnO/SR 2 −28.03 4.96 2.54 [55] CNT@NiO/NR 1.5 −24.7 4.24 1.05 [57] SCF@NiFe2O4/EP 4 −20.7 2.1 1.03 [58] BCN/NR 1.4 −54.24 4.16 0.28 [59] TiO2@C-Ni/CNTs/NR 1.8 −25.6 5.5 0.25 [60] BN@C/Ag/SR 2.3 − 6.89 0.42 [61] CNF@C-Ni/EP 2.2 −20.9 5.68 0.5 [62] SiCnw/BN/EP 3 −21.5 2.8 2.21 [63] MDCF@BN/EP 3 −52.77 5.6 0.99 [64] PVDF/Co/MXene composite foams 4 −45.6 2 1.36 [65] NiCo2O4/graphene oxide/carbon fibers elastomer 2.4 −55.15 8.25 15.55 [66] Notes: RL is the reflection loss; EABmax is the maximum effective absorption bandwidth; λ is the thermal conductivity; EG is the exfoliated graphite; EP is the epoxy resin; CIP is the carbonyl iron powder; SR is the silicone rubber; CNT is the Carbon nanotube; NR is the natural rubber; SCF is the short carbon fiber; BCN is the biomass-derived borocarbonitride; BN is the boron nitride; CNF is the carbon nanofiber; SiCnw is the silicon carbide nanowires; MDPF is the melamine derived carbon foam; PVDF is the a polyvinylidene fluoride. -
[1] XIE Y X, GUO Y Y, CHENG T, et al. Efficient electromagnetic wave absorption performances dominated by exchanged resonance of lightweight PC/Fe3O4@PDA hybrid nanocomposite[J]. Chemical Engineering Journal, 2023, 457: 14120. [2] HE Z Z, SUN R, XU H X, et al. Metal-organic-frameworks derived hollow carbon derivatives: Controllable configurations and optimized microwave absorption[J]. Carbon, 2024, 219: 118853. doi: 10.1016/j.carbon.2024.118853 [3] TANG Z M, XU L, XIE, et al. Synthesis of CuCo2S4@Expanded Graphite with crytal/amorphous heterointerface and defects for electromagnetic wave absorption[J]. Nature Communications, 2023, 14: 5951. doi: 10.1038/s41467-023-41697-6 [4] HUANG M, WANG Z Q, KONG N Z, et al. Vertically aligned and conformal BN-coated carbon fiber to achieve enhanced thermal conductivity and electrical insulation of a thermal interface material[J]. Chemical Engineering Journal, 2024, 490: 151621. doi: 10.1016/j.cej.2024.151621 [5] LI M H, LI L H, CHEN Y P, et al. Epoxy composite with metal-level thermal conductivity achieved by synergistic effect inspired by lamian noodles[J]. Composites Science and Technology, 2022, 228: 109677. doi: 10.1016/j.compscitech.2022.109677 [6] LI A, ZHANG C, ZHANG Y F, et al. Thermal conductivity of graphene-polymer composites: Mechanisms, properties, and applications[J]. Polymers, 2017, 9(9): 437. doi: 10.3390/polym9090437 [7] KUANG Z, CHEN Y, LU Y, et al. Fabrication of Highly Oriented Hexagonal Boron Nitride Nanosheet/Elastomer Nanocomposites with High Thermal Conductivity[J]. Small, 2015, 11(14): 1655-1659. doi: 10.1002/smll.201402569 [8] 贾琨, 王喆, 王蓬, 等. 导热吸波材料的研究进展及未来发展方向[J]. 材料导报, 2021, 35(9): 9133-9139+9149. doi: 10.11896/cldb.20020002JIA Kun, WANG Zhe, WANG Peng, et al. Research progress and future development direction of heat-conducting and wave-absorbing materials[J]. Materials Reports, 2021, 35(9): 9133-9139+9149 (in Chinese). doi: 10.11896/cldb.20020002 [9] DONG S, LIN C, MENG X. One-pot synthesis and microwave absorbing properties of ultrathin SrFe12O19 nanosheets[J]. Journal of Alloys and Compounds, 2019, 783: 779-784. doi: 10.1016/j.jallcom.2018.12.265 [10] YANG R B, REDDY P M, CHANG C J, et al. Synthesis and characterization of Fe3O4/polpyrrole/carbon nanotube composites with tunable microwave absorption properties: role of carbon nanotube and polypyrrole content[J]. Chemical Engineering Journal, 2016, 285: 497-507. doi: 10.1016/j.cej.2015.10.031 [11] ZHONG X C, ZHANG H N, HE N, et al. Superior microwave absorption properties of carbon-coated FeSiCr micro flakes[J]. Journal of Alloys and Compounds, 2024, 989: 174384. doi: 10.1016/j.jallcom.2024.174384 [12] GU Z F, YU Z C, HONG B, et al. Effect of lanthanum substitution on microstructures, magnetic properties and microwave absorption properties of SrCo2Z hexaferrites[J]. Journal of Alloys and Compounds, 2023, 969: 172296. doi: 10.1016/j.jallcom.2023.172296 [13] YU J A, LUO H, WANG Z H, et al. Surface morphology of magnetic carbon foam regulated electromagnetic properties for microwave absorption[J]. Journal of Alloys and Compounds, 2024, 971: 1727571. [14] KHANAHMADI S, MASOUDPANAH S M. Preparation and microwave absorption properties of CoFe2O4/NiCo2O4 composite powders[J]. Ceramics International, 2024, 50(6): 9779-9788. doi: 10.1016/j.ceramint.2023.12.299 [15] JIA Y, LI K, XUE L, et al. Mechanical and electromagnetic shielding performance of carbon fiber reinforced multilayered (PyC-SiC)n matrix composites[J]. Carbon, 2017, 111: 299-308. doi: 10.1016/j.carbon.2016.10.004 [16] LIU X, LU X L, GUAN H J, et al. Rational design of ZnO/ZnO nanocrystal-modified rGO foam composites with wide-frequency microwave absorption properties[J]. Ceramics International, 2021, 47(23): 33584-33595. doi: 10.1016/j.ceramint.2021.08.268 [17] WEN B, YANG G R, ZHOU X Y, et al. Intelligent diffusion regulation induced in-situ growth of cobalt nanoclusters on carbon nanotubes for excellent electromagnetic wave absorption[J]. Journal of Colloid and Interface Science, 2023, 634: 74-85. doi: 10.1016/j.jcis.2022.12.060 [18] PANG H F, DUAN Y P, HUANG L X, et al. Research advances in composition, structure and mechanisms of microwave absorbing materials[J]. Composites Part B: Engineering, 2021, 224: 109173. doi: 10.1016/j.compositesb.2021.109173 [19] LU J Y, XU L, XIE C, et al. Porous-multilayered Ti3C2Tx MXene hybrid carbon foams for tunable and efficient electromagnetic wave absorption[J]. Carbon, 2024, 229: 119477. doi: 10.1016/j.carbon.2024.119477 [20] CAO K Y, YE W P, ZHANG Y, et al. Fabrication of 3D micro-flower structured BiFeO3 growing on rGO nanosheets for enhanced electromagnetic wave absorption[J]. Chemical Engineering Journal, 2024, 496: 154184. doi: 10.1016/j.cej.2024.154184 [21] XU Z Z, ZHANG K, LI Y T, et al. Synthesis of SiC nanowires wrapped in trimetallic layered double hydroxide nanosheets with core-shell structure via self-assembly growth approach for effective electromagnetic wave absorption[J]. Journal of Alloys and Compounds, 2024, 976: 173316. doi: 10.1016/j.jallcom.2023.173316 [22] ZHANG H H, TANG J, ZHANG Y S, et al. Cross-scale construction of SiC-Si3N4 porous ceramics with superior electromagnetic wave absorption performance[J]. Ceramics International, 2024, 50(1): 2177-2184. doi: 10.1016/j.ceramint.2023.10.330 [23] XU Z H, HUANG J G, XIONG K W, et al. Tailoring electromagnetic wave absorption using in-situ precipitation of BaTiO3-BaFe12O19 glass ceramic[J]. Ceramics International, 2024, 50(2): 3119-3128. doi: 10.1016/j.ceramint.2023.11.061 [24] AYUB S, GUAN B H, AHMAD F, et al. Optimization of magnetite with modified graphene for microwave absorption properties[J]. Journal of Alloys and Compounds, 2023, 936: 168182. doi: 10.1016/j.jallcom.2022.168182 [25] ZHANG Y, LIU J, ZHANG Y, et al. Facile synthesis of hierarchical nanocomposites of aligned polyaniline nanorods on reduced graphene oxide nanosheets for microwave absorbing materials[J]. RSC Advances, 2017, 7(85): 54031-54038. doi: 10.1039/C7RA08794B [26] CHAO W, HAN X, PING X, et al. The electromagnetic property of chemically reduced graphene oxide and its application as microwave absorbing material[J]. Applied Physics Letters, 2011, 98(7): 217. [27] ZONG M, HUANG Y, ZHAO Y, et al. Facile preparation, high microwave absorption and microwave absorbing mechanism of RGO-Fe3O4 composites[J]. RSC Advances, 2013, 3(45): 23638-23648. doi: 10.1039/c3ra43359e [28] LI X, LI M, LU X, et al. A sheath-core shaped ZrO2-SiC/SiO2 fiber felt with continuously distributed SiC for broad-band electromagnetic absorption[J]. Chemical Engineering Journal, 2021, 419: 129414. doi: 10.1016/j.cej.2021.129414 [29] WALSER R M, WIN W, VALANJU P M. Shape-optimized ferromagnetic particles with maximum theoretical microwave susceptibility[J]. IEEE Transactions on Magnetics, 1998, 34(4): 1390-1392. doi: 10.1109/20.706558 [30] WENG X, LI B, ZHANG Y, et al. Synthesis of flake shaped carbonyl iron/reduced graphene oxide/polyvinyl pyrrolidone ternary nanocomposites and their microwave absorbing properties[J]. Journal of Alloys and Compounds, 2017, 695: 508-519. doi: 10.1016/j.jallcom.2016.11.083 [31] ADEBAYO L L, SOLEIMANI H, YAHYA N, et al. Recent advances in the development OF Fe3O4-BASED microwave absorbing materials[J]. Ceramics International, 2020, 46(2): 1249-1268. doi: 10.1016/j.ceramint.2019.09.209 [32] QING Y, ZHOU W, LUO F, et al. Microwave-absorbing and mechanical properties of carbonyl-iron/epoxy-silicone resin coatings[J]. Journal of Magnetism and Magnetic Materials, 2009, 321(1): 25-28. doi: 10.1016/j.jmmm.2008.07.011 [33] QUAN B, LIANG X H, XU G Y, et al. A permittivity regulating strategy to achieve high-performance electromagnetic wave absorbers with compatibility of impedance matching and energy conservation[J]. New Journal of Chemistry, 2017, 41(3): 1259-1266. doi: 10.1039/C6NJ03052A [34] QUAN B, LIANG X, JI G, et al. Dielectric polarization in electromagnetic wave absorption: Review and perspective[J]. Journal of Alloys and Compounds, 2017, 728: 1065-1075. doi: 10.1016/j.jallcom.2017.09.082 [35] CAO M S, SONG W L, HOU Z L, et al. The effects of temperature and frequency on the dielectric properties, electromagnetic interference shielding and microwave-absorption of short carbon fiber/silica composites[J]. Carbon, 2010, 48(3): 788-796. doi: 10.1016/j.carbon.2009.10.028 [36] YANG H J, CAO W Q, ZHANG D Q, et al. NiO Hierarchical Nanorings on SiC: Enhancing Relaxation to Tune Microwave Absorption at Elevated Temperature[J]. ACS applied materials & interfaces, 2015, 7(13): 7073-7077. [37] LI Z J, HOU Z L, SONG W L, et al. Unusual continuous dual absorption peaks in Ca-doped BiFeO3 nanostructures for broadened microwave absorption[J]. Nanoscale, 2016, 8(19): 10415-10424. doi: 10.1039/C6NR00223D [38] DU Y C, LIU W W, QIANG R, et al. Shell Thickness-Dependent Microwave Absorption of Core-Shell Fe3O4@C Composites[J]. ACS applied materials & interface, 2014, 6(15): 12997-13006. [39] SUN X, HE J, LI G, et al. Laminated magnetic graphene with enhanced electromagnetic wave absorption properties[J]. Journal of Materials Chemistry C, 2013, 1(4): 765-777. doi: 10.1039/C2TC00159D [40] ZHAI S, ZHANG P, XIAN Y, et al. Effective thermal conductivity of polymer composites: Theoretical models and simulation models[J]. International Journal of Heat and Mass Transfer, 2018, 117: 358-374. doi: 10.1016/j.ijheatmasstransfer.2017.09.067 [41] WU Y, YE K, LIU Z, et al. Cotton Candy-Templated Fabrication of Three-Dimensional Ceramic Pathway within Polymer Composite for Enhanced Thermal Conductivity[J]. ACS applied materials & interfaces, 2019, 11(47): 44700-44707. [42] HUANG X, JIANG P, TANAKA T. A Review of Dielectric Polymer Composites With High Thermal Conductivity[J]. IEEE Electrical Insulation Magazine, 2011, 27(4): 8-16. doi: 10.1109/MEI.2011.5954064 [43] SATO K, HORIBE H, SHIRAI T, et al. Thermally conductive composite films of hexagonal boron nitride and polyimide with affinity-enhanced interfaces[J]. Journal of Materials Chemistry, 2010, 20(14): 2749-2752. doi: 10.1039/b924997d [44] LUYT A S, MOLEFI J A, KRUMP H. Thermal, mechanical and electrical properties of copper powder filled low-density band linear low-density polyethylene composites[J]. Polymer Degradation and Stability, 2006, 91(7): 1629-1636. doi: 10.1016/j.polymdegradstab.2005.09.014 [45] KEMALOGLU S, OZKOC G, AYTAC A. Properties of thermally conductive micro and nano size boron nitride reinforced silicon rubber composites[J]. Thermochimica Acta, 2010, 499(1-2): 40-47. doi: 10.1016/j.tca.2009.10.020 [46] HUANG Z J, WANG R Q, JIANG W J, et al. Nacre-inspired flexible and thermally conductive phase change composites with parallelly aligned boron nitride nanosheets for advanced electronics thermal management[J]. Composites Science and Technology, 2024, 255: 110736. doi: 10.1016/j.compscitech.2024.110736 [47] WEI P, FENG L, Chen Q, et al. Highly thermally conductive composites with boron nitride nanoribbon array[J]. Chemical Engineering Journal, 2024, 488: 150915. doi: 10.1016/j.cej.2024.150915 [48] TANIMOTO M, YAMAGATA T, MIYATA K, et al. Anisotropic Thermal Diffusivity of Hexagonal Boron Nitride-Filled Polyimide Films: Effects of Filler Particle Size, Aggregation, Orientation, and Polymer Chain Rigidity[J]. ACS applied materials & interfaces, 2013, 5(10): 4374-4382. [49] GU J, MENG X, TANG Y, et al. Hexagonal boron nitride/polymethyl-vinyl siloxane rubber dielectric thermally conductive composites with ideal thermal stabilities[J]. Composites Part A, 2017, 92: 27-32. doi: 10.1016/j.compositesa.2016.11.002 [50] SONG S, WANG J, LIU C, et al. A facile route to fabricate thermally conductive and electrically insulating polymer composites with 3D interconnected graphene at an ultralow filler loading[J]. Nanoscale, 2019, 11(32): 15234-15244. doi: 10.1039/C9NR05153H [51] QIN Q, WEI D D, GAN J M, et al. Preparation of environmental-benign castor oil-derived polyurethane thermal conductive structural adhesives with superior strength[J]. Polymer, 2024, 298: 126831. doi: 10.1016/j.polymer.2024.126831 [52] YUAN H, WANG Y, LI T, et al. Fabrication of thermally conductive and electrically insulating polymer composites with isotropic thermal conductivity by constructing a three-dimensional interconnected network[J]. Nanoscale, 2019, 11(23): 11360-11368. doi: 10.1039/C9NR02491C [53] QIN M M, XU Y X, CAO R, et al. Efficiently Controlling the 3D Thermal Conductivity of a Polymer Nanocomposite via a Hyperelastic Double-Continuous Network of Graphene and Sponge[J]. Advanced Functional Materials, 2018, 28(45): 1805053. doi: 10.1002/adfm.201805053 [54] SINGH S K, AKHTAR M J, KAR K K. Impact of Al2O3, TiO2, ZnO and BaTiO3 on the microwave absorption properties of exfoliated graphite/epoxy composites at X-band frequencies[J]. Composites Part B, 2019, 167: 135-46. doi: 10.1016/j.compositesb.2018.12.012 [55] 汪旭文, 覃维, 王鲜, 等. 片状羰基铁粉/ZnO晶须填充聚合物基复合材料导热与吸波性能[J]. 磁性材料及器件, 2022, 53(4): 11-16.Wang Xuwen, Jia Wei, Wang Xian. Thermal conductivity and wave-absorption properties of polymer matrix composites filled with flake carbonyl iron powder/ZnO whiskers[J]. Journal of Magnetic Materials and Devices, 2022, 53(4): 11-16 (in Chinese). [56] 郑凯, 贾琨, 刘伟, 等. 导热吸波硅橡胶的功能特性及应用研究[J]. 化工新型材料, 2020, 48(6): 77-80+84.Zheng Kai, Jia Kun, Liu Wei, et al. Functional properties and applications of thermally conductive and wave-absorbing silicone rubber[J]. New Chemical Materials, 2020, 48(6): 77-80+84 (in Chinese). [57] ZHOU M F, WAN G P, MOU P P, et al. CNT@NiO/natural rubber with excellent impedance matching and low interfacial thermal resistance toward flexible and heat-conducting microwave absorption applications[J]. Journal of Materials Chemistry C, 2021, 9(3): 869-880. doi: 10.1039/D0TC04388E [58] LIU H B, FU R L, SU X Q, et al. SCF-NiFe2O4/epoxy composites with high thermal conductivity and electromagnetic interference resistance[J]. Journal of Materials Science: Materials in Electronics, 2020, 31: 21325-21334. doi: 10.1007/s10854-020-04644-5 [59] MOU P P, ZHAO J C, WANG G Z, et al. BCN nanosheets derived from coconut shells with outstanding microwave absorption and thermal conductive properties[J]. Chemical Engineering Journal, 2022, 437: 135285. doi: 10.1016/j.cej.2022.135285 [60] WU L H, LIU X, WAN G P, et al. Ni/CNTs and carbon coating engineering to synergistically optimize the interfacial behaviors of TiO2 for thermal conductive microwave absorbers[J]. Chemical Engineering Journal, 2022, 448: 137600. doi: 10.1016/j.cej.2022.137600 [61] LUO C, FEI D Z, ZHOU J T, et al. Silver particle-modified BN@C composites for thermal management and microwave absorption[J]. Ceramics International, 2024, 50(13PA): 22252-22265. [62] QIAN Y X, TAO Y, LI W, et al. High electromagnetic wave absorption and thermal management performance in 3D CNF@C-Ni/epoxy resin composites[J]. Chemical Engineering Journal, 2021, 425: 131608. doi: 10.1016/j.cej.2021.131608 [63] PAN D, YANG G, ABO-DIEF H M, et al. Vertically Aligned Silicon Carbide Nanowires /Boron Nitride Cellulose Aerogel Networks Enhanced Thermal Conductivity and Electromagnetic Absorbing of Epoxy Composites[J]. Nano-Micro Letters, 2022, 14: 118. doi: 10.1007/s40820-022-00863-z [64] QIAN Y X, TAO Y, LI Y, et al. High performance epoxy resin with efficient electromagnetic wave absorption and heat dissipation properties for electron packaging by modification of 3D MDCF@hBN[J]. Chemical Engineering Journal, 2022, 441: 136033. doi: 10.1016/j.cej.2022.136033 [65] LI R S, GAO Q, XIN H N, et al. Lightweight, multifunctional MXene/polymer composites with enhanced electromagnetic wave absorption and high-performance thermal conductivity[J]. Carbon, 2021, 183: 301-312. doi: 10.1016/j.carbon.2021.07.029 [66] ZHANG Z, WANG J D, SHANG J, et al. A Through-Thickness Arrayed Carbon Fibers Elastomer with Horizontal Segregated Magnetic Network for Highly Efficient Thermal Management and Electromagnetic Wave Absorption[J]. Small, 2022, 19(4): 2205716. -
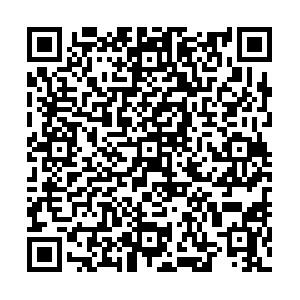
计量
- 文章访问数: 37
- HTML全文浏览量: 51
- 被引次数: 0