Progress on chain-like electromagnetic wave absorption materials with core-shell structures
-
摘要: 电磁波吸收材料不仅可以解决电磁污染、电磁干扰、电磁泄露等问题,还是有效的雷达隐身材料,因而吸引了广大研究者的热忱。核壳式链状电磁复合材料作为新型的电磁波吸收材料,表现出多重的结构优势。介电壳层与磁性内核的复合能够产生电磁损耗协同作用;高长径比的一维结构,提供了电磁波的传输路径;自组装形成的三维网络,增强了电磁波的多重反射;类天线效应有助于增加电磁波的多重散射;此外,选择恰当的介电壳层能够使核壳式链状电磁复合吸波材料兼顾抗氧化性、耐腐蚀性、耐高温性等,确保了电磁波吸收材料的环境适应性。根据现阶段的研究进展,本文系统综述了核壳式链状电磁复合吸波材料的制备方法,对比分析了长径比、壳层类型、壳层厚度、壳层数量、多孔结构以及壳层的晶相组成等结构因素对吸波性能的影响,阐明了核壳式链状电磁复合吸波材料的详细损耗机制,展望了核壳式链状电磁复合吸波材料的改进策略与发展方向。Abstract: Electromagnetic wave absorbing materials can not only solve the problems of electromagnetic pollution, electromagnetic interference and electromagnetic leakage, but also are effective radar stealth materials, thus attracting the enthusiasm of researchers. As a new type of electromagnetic wave absorbing materials, core-shell chain electromagnetic composites show multiple structural advantages. The composite of dielectric shell layer and magnetic core can produce electromagnetic loss synergy; the one-dimensional structure with high aspect ratio provides the transmission path of electromagnetic wave; the three-dimensional network formed by self-assembly enhances the multiple reflections of electromagnetic wave; the antenna-like effect helps to increase the multiple scattering of electromagnetic wave; in addition, choosing the proper dielectric shell layer can make the core-shell chain electromagnetic composite absorbing material take into account the antioxidant property, the corrosion resistance, the high temperature resistance, etc., which ensures that the core-shell chain electromagnetic composite absorbing material has a good resistance to oxidation, corrosion and high temperature, high temperature resistance, etc., which ensures the environmental adaptability of electromagnetic wave absorbing materials. According to the research progress at this stage, this paper systematically reviews the preparation method of core-shell chain electromagnetic composite wave-absorbing materials, comparatively analyzes the influence of structural factors such as aspect ratio, shell layer type, shell layer thickness, number of shell layers, porous structure and crystalline phase composition of the shell layer on the wave-absorbing performance, elucidates the detailed loss mechanism of core-shell chain electromagnetic composite wave-absorbing materials, and prospects for improvement strategy and development direction of core-shell chain electromagnetic composite wave-absorbing materials. Improvement strategy and development direction of core-shell chain electromagnetic composite wave-absorbing materials.
-
图 3 SEM图:(a)化学还原法制备的Ni@PANI纳米链[48]; (b)和(c)表面活性剂诱导法制备的Ni纳米链[20];(d)溶剂热法制备出的α-Fe@Fe3O4纳米链[23]:(e)磁诱导自组装法制备出Yolk–shell型Fe3O4@C(掺杂N)纳米链[29]。
Figure 3. SEM images: (a) Ni@PANI nanochains prepared by chemical reduction[48]; (b) and (c) Ni nanochains prepared by surfactant-induced method[20]; (d) α-Fe@Fe3O4 nanochains prepared by solvothermal method[23]; (e) Yolk-shell type Fe3O4@C (N-doped) nanochains prepared by magnetic-induced self-assembly[29].
表 1 不同吸波剂性能对比
Table 1. Comparison of Performance of Different Absorber
Samples Filling ratio/wt% RL min/dB EAB/GHz Thickness/mm Ref Ni@PANI − −51.16 3.4 2.7 [48] 1 D@2 D Fe − −57.3 11.5 1.9 [17] Ni@PVP 50 −49 4.2 1.8 [20] α-Fe@Fe3O4 − −25.6 3.6(14.4~18.0) 0.8 [23] Yolk–shell Fe3O4@C 20 −63.09 5.34(9.62 ~14.96) 3.1 [29] Fe@Fe3C@C −58.0 3.5 2.4 [30] Co@PANI − −73.16 4.98(12.28~17.26) 4.63 [36] Ni@PANI − −65.06 5.02 3.88 [36] FeCo/PVP 50 −20 2.0−10.0 2.0−8.0 [31] CoNi/PVDF 60 −48.99 3.5(13~16.5) 5.0 [32] TiO2@Fe3O4@PPy − −61.8 6.0(10.8 ~16.8) 3.3 [15] Yolk-Shell Fe3O4@void@SiO2 35 −54.2 5.9(11.49~17.39) 1.8 [39] ZnFe2O4@SiO2@C @NiCo2O4 30 −54.29 5.66(11.94~17.60) 2.39 [41] Fe3O4@void@mSiO2@ MnO2 40 −45.76 5.13(10.49 ~15.62) 6.1 [44] Fe3O4@TiO2 − −21.29 5.09(11.83~16.92) 7.0 [47] Fe3O4@C − −45.3 2.3 GHz(7.8~ 11.1 GHz) 2.2 [51] Notes: RL min−Minimum reflection loss; EAB−Effective absorption bandwidth; PANI−Polyaniline; PVP−Polyvinyl pyrrolidone; PVDF−Polyvinylidene fluoride; PPy−Polypyrrole. -
[1] 赵天际. 吸波材料的研究进展与展望[D]. 哈尔滨工业大学, 2021.ZHAO, T. J. (2021). Research progress and prospects of wave-absorbing materials [D]. Harbin Institute of Technology. (in Chinese) [2] LV H, YANG Z, PAN H, et al. Electromagnetic absorption materials: Current progress and new frontiers[J]. Progress in Materials Science, 2022, 127: 100946. doi: 10.1016/j.pmatsci.2022.100946 [3] PEYMANFAR R, JAVANSHIR S, NAIMI-JAMAL M R, et al. Morphology and medium influence on microwave characteristics of nanostructures: A review[J]. Journal of Materials Science, 2021, 56(31): 17457-17477. doi: 10.1007/s10853-021-06394-z [4] WANG Y FAN, ZHU L, HAN L, et al. Recent Progress of One-Dimensional Nanomaterials for Microwave Absorption: A Review[J]. ACS Applied Nano Materials, 2023, 6(9): 7107-7122. doi: 10.1021/acsanm.3c00818 [5] HANG T, ZHENG J, ZHENG Y, et al. Wheat-like Ni-coated core–shell silver nanowires for effective electromagnetic wave absorption[J]. Journal of Colloid and Interface Science, 2023, 649: 394-402. doi: 10.1016/j.jcis.2023.06.087 [6] DAS B, MANDAL K, SEN P, et al. Effect of aspect ratio on the magnetic properties of nickel nanowires[J]. Journal of Applied Physics, 2008, 103(1): 013908. doi: 10.1063/1.2828026 [7] 李文婷. 一维磁性吸波材料的制备及吸波性能研究[D]. 青岛理工大学, 2019.LI, W. T. (2019). Preparation and absorption properties study of one-dimensional magnetic wave-absorbing materials [D]. Qingdao University of Technology. (in Chinese) [8] LUO Z, ZHANG X A, EVANS B A, et al. Active Periodic Magnetic Nanostructures with High Aspect Ratio and Ultrahigh Pillar Density[J]. ACS Applied Materials & Interfaces, 2020, 12(9): 11135-11143. [9] 童周禹. 一维磁性花状复合材料的制备及吸波性能研究[D]. 青岛理工大学, 2021.TONG, Z. Y. (2021). Preparation and absorption performance study of one-dimensional magnetic flower-like composite materials [D]. Qingdao University of Technology. (in Chinese) [10] REN L, WANG Y, ZHANG X, et al. Efficient microwave absorption achieved through in situ construction of core-shell CoFe2O4@mesoporous carbon hollow spheres[J]. International Journal of Minerals, Metallurgy and Materials, 2023, 30(3): 504-514. doi: 10.1007/s12613-022-2509-1 [11] 郝慧敏, 王黎明, 曹刘奇, 等. 核壳结构吸波材料的研究进展[J]. 化工新型材料, 2022, 50(6): 14-18.HAO, H M, WANG, L M, CAO, L Q, et al. (2022). Research progress of core-shell structured wave-absorbing materials[J]. New Chemical Materials, 50(6): 14-18. (in Chinese) [12] GAI L, ZHAO H, WANG F, et al. Advances in core—shell engineering of carbon-based composites for electromagnetic wave absorption[J]. Nano Research, 2022, 15(10): 9410-9439. doi: 10.1007/s12274-022-4695-6 [13] ZENG X, CHENG X, YU R, et al. Electromagnetic microwave absorption theory and recent achievements in microwave absorbers[J]. Carbon, 2020, 168: 606-623. doi: 10.1016/j.carbon.2020.07.028 [14] 王士鹏. 一维核壳结构四氧化三铁基复合材料的制备及其吸波性能研究[D]. 淮北师范大学, 20202023-10-04]. WANG, S. P. (2020). Preparation and absorption performance study of one-dimensional core-shell structured ferrite-based composite materials [D]. Huaibei Normal University. Retrieved October 4, 2023. (in Chinese) [15] DING J, WANG L, ZHAO Y, et al. Boosted Interfacial Polarization from Multishell TiO2@Fe3O4@PPy Heterojunction for Enhanced Microwave Absorption[J]. Small, 2019, 15(36): 1902885. doi: 10.1002/smll.201902885 [16] MUANGNAPOH T, SRIMARA P, VAS-UMNUAY P. Template- and Magnetic Field-Free Chemical Reduction of Ni Nanochains for Ni–Al2O3Cermet Films: Growth Control, Characterization, and Application[J]. ACS Omega, 2020, 5(38): 24584-24591. doi: 10.1021/acsomega.0c03077 [17] LU L, AI Z, LI J, et al. Synthesis and characterization of Fe− Fe2O3 core− shell nanowires and nanonecklaces[J]. Crystal growth & design, 2007, 7(2): 459-464. [18] SHI X, YOU W, LI X, et al. In-situ regrowth constructed magnetic coupling 1D/2D Fe assembly as broadband and high-efficient microwave absorber[J]. Chemical Engineering Journal, 2021, 415: 128951. doi: 10.1016/j.cej.2021.128951 [19] SREEDHALA S, VINOD C P. Surfactant assisted formation of ruthenium nanochains under mild conditions and their catalytic CO oxidation activity[J]. Chemical Communications, 2015, 51(50): 10178-10181. doi: 10.1039/C4CC09430A [20] LIU J, CAO M S, LUO Q, et al. Electromagnetic Property and Tunable Microwave Absorption of 3D Nets from Nickel Chains at Elevated Temperature[J]. ACS Applied Materials & Interfaces, 2016, 8(34): 22615-22622. [21] OZDEMIR T, SANDAL D, CULHA M, et al. Assembly of magnetic nanoparticles into higher structures on patterned magnetic beads under the influence of magnetic field[J]. 2010. [22] SAHOO A, DAS M, MANDAL P, et al. Hydrothermal synthesis of Bi2Fe4O9 nanochains and study of their multiferroic coupling[J]. Materials Letters, 2021, 296: 129905. doi: 10.1016/j.matlet.2021.129905 [23] WANG S, ZHANG M, LIU Q, et al. Synthesis of chain-like ɑ-Fe/Fe3O4 core/shell composites exhibiting enhanced microwave absorption performance in high-frequency under an ultrathin matching thickness[J]. Journal of Materials Science: Materials in Electronics, 2018, 29(24): 21040-21050. doi: 10.1007/s10854-018-0250-3 [24] 苗腾. 磁性一维复合纳米材料的设计、合成及其性能研究[D]. 上海工程技术大学, 2019.MIAO, T. (2019). Design, synthesis, and performance study of magnetic one-dimensional composite nanomaterials [D]. Shanghai University of Engineering Science. [25] ZHANG T, YUE Q, PAN P, et al. One-dimensional nanochains consisting of magnetic core and mesoporous aluminosilicate for use as efficient nanocatalysts[J]. Nano Research, 2021, 14(11): 4197-4203. doi: 10.1007/s12274-021-3383-2 [26] BEHERA M, KUMARI N, RAZA K, et al. Fabrication of glutathione functionalized self-assembled magnetite nanochains for effective removal of crystal violet and phenol red dye from aqueous matrix[J]. Environmental Science and Pollution Research, 2022, 29(48): 72260-72278. doi: 10.1007/s11356-022-19520-4 [27] KO P J, TAKAMURA T, SANDHU A. Magnetically Induced Self-Assembly of Superparamagnetic Particles for Medical Diagnostics[J]. Journal of the Japan Society of Powder and Powder Metallurgy, 2014, 61(S1): S111-S116. doi: 10.2497/jjspm.61.S111 [28] SU X, LIU Y, LIAO Z, et al. A review of 1D magnetic nanomaterials in microwave absorption[J]. Journal of Materials Science, 2023, 58(2): 636-663. doi: 10.1007/s10853-022-08054-2 [29] MINGTAO QIAO, XINGFENG LEI, YONG MA, et al. Application of yolk–shell Fe3O4@N-doped carbon nanochains as highly effective microwave-absorption material[J]. Nano Research, 2018. [30] SUN J C, HE Z, DONG W, et al. Broadband and strong microwave absorption of Fe/Fe3C/C core–shell spherical chains enhanced by dual dielectric relaxation and dual magnetic resonances[J]. Journal of Alloys and Compounds, 2019, 782: 193-202. doi: 10.1016/j.jallcom.2018.12.198 [31] ZHANG X, LI Y, LIU R, et al. High-Magnetization FeCo Nanochains with Ultrathin Interfacial Gaps for Broadband Electromagnetic Wave Absorption at Gigahertz[J]. ACS Applied Materials & Interfaces, 2016, 8(5): 3494-3498. [32] QIAO M, LI J, LI S, et al. Hierarchical CoNi alloys toward microwave absorption application: Chain-like versus particle-like[J]. Journal of Alloys and Compounds, 2022, 926: 166854. doi: 10.1016/j.jallcom.2022.166854 [33] ZHAO B, LI Y, ZENG Q, et al. Galvanic Replacement Reaction Involving Core–Shell Magnetic Chains and Orientation-Tunable Microwave Absorption Properties[J]. Small, 2020, 16(40): 2003502. doi: 10.1002/smll.202003502 [34] ZHOU W, CHEN W, NAI J, et al. Selective Synthesis of Peapodlike Ni/Ni3S2 Nanochains and Nickel Sulfide Hollow Chains and Their Magnetic Properties[J]. Advanced Functional Materials, 2010, 20(21): 3678-3683. doi: 10.1002/adfm.201001287 [35] YOU W, PEI K, YANG L, et al. In situ dynamics response mechanism of the tunable length-diameter ratio nanochains for excellent microwave absorber[J]. Nano Research, 2020, 13(1): 72-78. doi: 10.1007/s12274-019-2574-6 [36] DAI B, MA Y, FENG S, et al. Fabrication of one-dimensional M (Co, Ni)@polyaniline nanochains with adjustable thickness for excellent microwave absorption properties[J]. Journal of Colloid and Interface Science, 2022, 627: 113-125. doi: 10.1016/j.jcis.2022.06.137 [37] KOTHANDARAMAN R K, JIANG Y, FEURER T, et al. Near-Infrared-Transparent Perovskite Solar Cells and Perovskite-Based Tandem Photovoltaics[J]. Small Methods, 2020, 4(10): 2000395. doi: 10.1002/smtd.202000395 [38] PRÉAULT V, CORCOLLE R, DANIEL L, et al. Influence of skin effect on the effective shielding effectiveness of composite materials[J]. Journal of Applied Physics, 2014, 115(15): 154904. doi: 10.1063/1.4871197 [39] MINGTAO QIAO, DAN WEI, XIAOWEI HE, et al. Novel yolk–shell Fe3O4@void@SiO2@PPy nanochains toward microwave absorption application[J]. Journal of Materials Science, 2020. [40] PAN’KOV A A. Maxwell–Wagner relaxation in fibrous polydisperse magnetoelectric piezocomposites[J]. Mechanics of Composite Materials, 2013, 49(1): 45-50. doi: 10.1007/s11029-013-9319-5 [41] MA M, LI W, TONG Z, et al. NiCo2O4 nanosheets decorated on one-dimensional ZnFe2O4@SiO2@C nanochains with high-performance microwave absorption[J]. Journal of Colloid and Interface Science, 2020, 578: 58-68. doi: 10.1016/j.jcis.2020.05.044 [42] LI Y, LIU X, LIU R, et al. Improved microwave absorption properties by atomic-scale substitutions[J]. Carbon, 2018, 139: 181-188. doi: 10.1016/j.carbon.2018.06.030 [43] LIU Q, ZHANG D, FAN T. Electromagnetic wave absorption properties of porous carbon/Co nanocomposites[J]. Applied Physics Letters, 2008, 93(1): 013110. doi: 10.1063/1.2957035 [44] QIAO M, LI J, WEI D, et al. Chain-like Fe3O4@void@mSiO2@MnO2 composites with multiple porous shells toward highly effective microwave absorption application[J]. Microporous and Mesoporous Materials, 2021, 314: 110867. doi: 10.1016/j.micromeso.2020.110867 [45] CHENG J B, SHI H G, CAO M, et al. Porous carbon materials for microwave absorption[J]. Materials Advances, 2020, 1(8): 2631-2645. doi: 10.1039/D0MA00662A [46] FANG Y, XUE W, ZHAO R, et al. Effect of nanoporosity on the electromagnetic wave absorption performance in a biomass-templated Fe3O4 /C composite: a small-angle neutron scattering study[J]. Journal of Materials Chemistry C, 2020, 8(1): 319-327. doi: 10.1039/C9TC04569D [47] QIAO M, WANG J, WEI D, et al. Influence of crystalline phase evolution of shells on microwave absorption performance of core-shell Fe3O4@TiO2 nanochains[J]. Materials Today Nano, 2022, 18: 100203. doi: 10.1016/j.mtnano.2022.100203 [48] LI Y, WANG J, LI H, et al. Effect of conductive PANI vs. insulative PS shell coated Ni nanochains on electromagnetic wave absorption[J]. Journal of Alloys and Compounds, 2020, 821: 153531. doi: 10.1016/j.jallcom.2019.153531 [49] WANG J, OR S W, TAN J. Enhanced microwave electromagnetic properties of core/shell/shell-structured Ni/SiO2/polyaniline hexagonal nanoflake composites with preferred magnetization and polarization orientations[J]. v Materials & Design, 2018, 153: 190-202. [50] YAN L, WANG J, HAN X, et al. Enhanced microwave absorption of Fe nanoflakes after coating with SiO2 nanoshell[J]. Nanotechnology, 2010, 21(9): 095708. doi: 10.1088/0957-4484/21/9/095708 [51] WANG S, HUANG F, ZHANG M, et al. Fe3O4/carbon chain-like core/shell composites: Synthesis and microwave absorption properties[J]. Integrated Ferroelectrics, 2018[2024-07-26]. [52] QIAO M, TIAN Y, LI J, et al. Core-shell Fe3O4@SnO2 nanochains toward the application of radar-infrared-visible compatible stealth[J]. Journal of Colloid and Interface Science, 2022, 609: 330-340. doi: 10.1016/j.jcis.2021.12.012 -
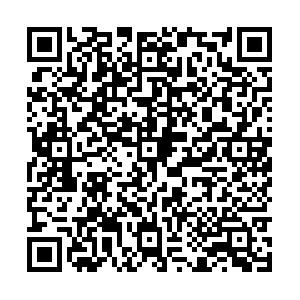
计量
- 文章访问数: 25
- HTML全文浏览量: 34
- 被引次数: 0