DC conduction liquid metal electromagnetic pump flow field distortion and its electromagnetic configuration compensation
-
摘要: 利用直流传导电磁泵(DC-EMP)驱动低熔点的镓基液态金属作为冷却工质的散热方法,在高热流芯片的热控领域展现出重要的应用前景。为削弱液态金属的磁流体动力学(Magnetohydrodynamic, MHD)效应及其导致的流场畸变,提高DC-EMP的驱动性能。对液态金属的流动特性,流道内电流密度场、磁感应强度及洛伦兹力矢量分布进行了研究,采用磁轭和绝缘板进行电磁构型补偿以提高DC-EMP作用区的磁感应强度和有效电流,从而削弱MHD效应。结果表明:受MHD效应的影响,靠近侧壁面的液态金属被加速主流区流速降低,流道内液态金属在穿过作用区后会发生严重的流场畸变。液态金属切割磁感线产生的感应电流在作用区端部形成涡电流,减弱了作用区内的有效电流,同时在涡电流和电、磁场端部效应的耦合下,在作用区外产生与流速方向相反的洛伦兹力阻力,抑制液态金属进入和离开作用区,进而降低DC-EMP的性能。试验表明电磁泵的进出口压差∆P随输入电流It的增加而增大,当It=50 A时补偿后的∆P较传统结构提高了78.08%。
-
关键词:
- 镓基液态金属复合材料 /
- 直流传导电磁泵 /
- 电磁构型补偿 /
- 芯片散热 /
- 热管理
Abstract: A heat dissipation method using a DC-conduction electromagnetic pump (DC-EMP) to drive a low-melting-point gallium-based liquid metal as a cooling workpiece, which has significant prospects in the field of heat dissipation in chips. In order to weaken the magnetohydrodynamic (MHD) effect of liquid metals and its resulting flow field distortion, and to improve the performance of DC-EMP. The flow characteristics of liquid metal, current density field, magnetic induction strength and Lorentz force vector distribution in the flow channel were investigated, and the electromagnetic configuration was compensated by using an iron yoke and an insulation bars in order to increase the magnetic induction strength and the effective current in the DC-EMP region of action, thus weakening the MHD effect. The results show that the liquid metal near the side wall surface is accelerated by the MHD effect, the flow velocity in the main flow zone decreases, and the liquid metal in the flow channel undergoes serious flow field distortion after passing through the magnetic field zone. The induced current generated by the liquid metal cutting the magnetic susceptibility line forms an eddy current at the end of the action zone, which attenuates the effective current within the action zone, and at the same time, under the coupling of the eddy current and the end effect of electric and magnetic fields, it generates a Lorentz force resistance outside the action zone in the opposite direction of the flow velocity, which inhibits the liquid metal from entering and leaving the action zone, and thus reduces the performance of the DC-EMP. The tests show that the ∆P of the electromagnetic pump increases with the increase of the input current It, and the compensated ∆P is improved by 78.08% compared with the conventional structure when It = 50 A. -
表 1 Ga68In20Sn12和水的物性参数
Table 1. Physical parameters of Ga68In20Sn12 and water
Parameters Ga68In20Sn12 Water Electrical conductivity/
(106S·m−1)3.6 <5×10−4 Density/(kg·m−3) 6050 998 Dynamic viscosity/(mPa·s) 2.40 0.97 Coefficient of thermal
conductivity/(W·m−1·K−1)23.67 0.61 表 2 材料物性参数
Table 2. Physical parameters
Element Materials Relative magnetic
permeabilityElectrical
conductivity/(S·m−1)Relative electric
permittivityDensity/
(kg·m−3)Dynamic
viscosity/(Pa·s)Magnet Sm2Co17 1.1 0 1 8300 - Electrode Copper 1 5.99×107 1 8940 - Channel PLA 1 0 1 1260 - Liquid metal Ga68In20Sn12 1 3.6×106 1 6050 2.4×10−3 表 3 网格无关性验证
Table 3. Mesh independence verification
Number of meshes Δδmin/mm Pressure/Pa Relative Error M1 990248 0.379 2954.6 1.22% M2 1118999 0.142 2973.8 0.55% M3 1476318 0.059 2990 - Notes: Δδmin—Mesh thickness -
[1] MUHAMMAD A, SELVAKUMAR D, WU J. Numerical investigation of laminar flow and heat transfer in a liquid metal cooled mini-channel heat sink[J]. International Journal of Heat and Mass Transfer, 2020, 150: 119265. doi: 10.1016/j.ijheatmasstransfer.2019.119265 [2] 李锡英, 王爽, 鲁璐, 等. 液相夹杂复合软材料的设计、制备与力学性能研究进展[J]. 复合材料学报, 2021, 38(1): 1-15.LI X Y, WANG S, LU L, et al. Design, fabrication and mechanical properties of soft composites with liquid inclusions[J]. Acta Materiae Compositae Sinica, 2021, 38(1): 1-15(in Chinese). [3] HEINZEL A, HERING W, KONYS J, et al. Liquid Metals as Efficient High-Temperature Heat-Transport Fluids[J]. Energy Technology, 2017, 5(7): 1026-1036. doi: 10.1002/ente.201600721 [4] KIM H R. The design and fabrication of a small MHD pump for liquid sodium circulation[J]. Annals of Nuclear Energy, 2014, 73: 162-167. [5] AL-HABAHBEH O M, AL-SAQQA M, Safi M, et al. Review of magnetohydrodynamic pump applications[J]. Alexandria Engineering Journal, 2016, 55(2): 1347-1358. doi: 10.1016/j.aej.2016.03.001 [6] KIM C N. Numerical examination of liquid metal magnetohydrodynamic flow in multiple channels in the plane perpendicular to the magnetic field[J]. Journal of Mechanical Science and Technology, 2014, 28(12): 4959-4968. doi: 10.1007/s12206-014-1117-z [7] 王军, 鹿鹏, 黄护林, 等. 垂直上升通道内气泡-液态金属MHD流动与传热的数值研究[J]. 中国电机工程学报, 2024, 43(3): 1058-1070.WANG J, LU P, HUANG H L, et al. Numerical Investigation on MHD Flow and Heat Transfer of Bubble-Liquid Metal in a Vertical Upward Channel[J]. Proceedings of the CSEE, 2024, 43(3): 1058-1070(in Chinese). [8] CHEN L, LI M, NI M, et al. MHD effects and heat transfer analysis in magneto-thermo-fluid-structure coupled field in DCLL blanket[J]. International Communications in Heat and Mass Transfer, 2017, 84: 110-120. doi: 10.1016/j.icheatmasstransfer.2017.04.009 [9] 王凡, 张秀杰, 潘传杰. 高特征参数下绝缘圆管内液态金属MHD效应研究[J]. 核聚变与等离子体物理, 2023, 43(2): 175-179.WANG F, ZHANG X J, PAN C J. Investigations on the MHD effect of liquid metals through an insulating circular pipe under large characteristic parameters[J]. Nuclear Fusion and Plasma Physics, 2023, 43(2): 175-179(in Chinese). [10] 雷天扬, 孟旭, 王增辉, 等. 强磁场下液态金属微槽道流动与换热实验研究[J]. 中国科学院大学学报, 2021, 38(4): 459-666. doi: 10.7523/j.issn.2095-6134.2021.04.004LEI T Y, MENG X, WANG Z H, et al. Experimental study of flow pattern and heat transfer of liquid metal in microchannel under the magnetic field[J]. Journal of University of Chinese Academy of Sciences, 2021, 38(4): 459-666(in Chinese). doi: 10.7523/j.issn.2095-6134.2021.04.004 [11] KANDEV N, KAGAN V, DAOUD A. Electromagnetic DC Pump of Liquid Aluminum: Computer Simulation and Experimental Study[J]. FDMP: Fluid Dynamics & Materials Processing, 2010, 6(3): 291-318. [12] ZHANG X D, ZHOU Y X, LIU J. A novel layered stack electromagnetic pump towards circulating metal fluid: Design, fabrication and test[J]. Applied Thermal Engineering, 2020, 179: 115610. doi: 10.1016/j.applthermaleng.2020.115610 [13] LEE G H, KIM H R. Design analysis of DC electromagnetic pump for liquid sodium–CO2 reaction experimental characterization[J]. Annals of Nuclear Energy, 2017, 109: 490-497. doi: 10.1016/j.anucene.2017.05.054 [14] 谢开旺, 刘明, 刘静, 等. 电磁泵驱动室温金属流体的数值模拟与试验研究[J]. 电子机械工程, 2009, 25(3): 1-5+23. doi: 10.3969/j.issn.1008-5300.2009.03.001XIE K W, LIU M, LIU J, et al. Simulation and experiment of study on electromagnetic pump for driving liquid metal[J]. Electro-Mechanical Engineering, 2009, 25(3): 1-5+23(in Chinese). doi: 10.3969/j.issn.1008-5300.2009.03.001 [15] JIAN B T, XI Z, JING L, et al. Gallium-Based Liquid Metal Amalgams: Transitional-State Metallic Mixtures (TransM2ixes) with Enhanced and Tunable Electrical, Thermal, and Mechanical Properties.[J]. ACS applied materials & interfaces, 2017, 9(41): 35977-35987. [16] LEE G H, KIM H R. Magnetic-field analysis of an MHD channel in a liquid-metal circulation system of a prototype GenIV sodium fast reactor[J]. Annals of Nuclear Energy, 2018, 115: 343-351. doi: 10.1016/j.anucene.2018.01.049 [17] SUN P, LIU C K, HE Z Z. A compact Double-spiral electromagnetic pump for liquid metal cooling[J]. Annals of Nuclear Energy, 2023, 180: 109486. doi: 10.1016/j.anucene.2022.109486 [18] LEE G H, KIM H R. Numerical analysis of the electromagnetic force for design optimization of a rectangular direct current electromagnetic pump[J]. Nuclear Engineering and Technology, 2018, 50(6): 869-876. doi: 10.1016/j.net.2018.04.010 [19] 王赵波, 陈龙, 倪明玖. 强磁场对突扩管中液态金属剪切层的影响[J]. 工程热物理学报, 2021, 42(10): 2672-2680.WANG Z B, CHEN L, NI M J. Influence of the High Magnetic Fields on the Shear Layer of Liquid Metal Sudden Expansion Flow[J]. Journal of Engineering Thermophysics, 2021, 42(10): 2672-2680(in Chinese). [20] HUNT J C R. Magnetohydrodynamic flow in rectangular ducts[J]. Journal of Fluid Mechanics, 1965, 21(4): 577-590. doi: 10.1017/S0022112065000344 [21] LEE G H, KIM H R. Mathematical approach for optimization of magnetohydrodynamic circulation system[J]. Nuclear Engineering and Technology, 2019, 51(3): 654-664. doi: 10.1016/j.net.2018.12.008 [22] KWAK J, KIM H R. Design and preliminary test of an annular linear induction electromagnetic pump for a sodium-cooled fast reactor thermal hydraulic experiment[J]. Journal of Nuclear Science and Technology, 2017, 54(12): 1292-1299. doi: 10.1080/00223131.2017.1365020 [23] YAO L, LI X B, ZHANG H N, et al. A novel Halbach array electromagnetic pump for liquid metal flow: Design proposal and performance analysis[J]. Annals of Nuclear Energy, 2023, 183: 109614. [24] TAKORABET N. Computation of force density inside the channel of an electromagnetic pump by Hermite projection[J]. IEEE Transactions on Magnetics, 2006, 42(3): 430-433. doi: 10.1109/TMAG.2005.863085 -
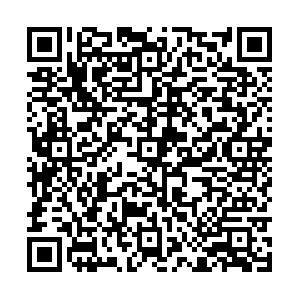
计量
- 文章访问数: 32
- HTML全文浏览量: 9
- 被引次数: 0