Electric field driven micro 3D printing of high-precision circuit on bismaleimide resin matrix composite
-
摘要: 纤维改性双马树脂基复合材料凭借其优异的力学性能、耐高温、耐腐蚀特性,被广泛应用于航空航天、智能蒙皮、共形天线、电磁屏蔽、高频电路基板、电加热等领域。然而,由于石英纤维增强双马树脂基复合材料具有非平整、异质、各向异性的特点,在此基材上简单、高效、低成本制造高分辨率微细电路是当前亟待解决的难题。本文提出了一种基于电场驱动微3D打印在石英纤维增强双马树脂基复合材料上制造高精度电路的新方法,阐述了基本成形原理和关键技术实现,探究了非平整异质复合材料表面电场分布特点和场强变化规律,提出通过调节电场强度阈值实现稳定打印的策略;通过实验揭示了主要工艺参数对制造电路精度、形貌以及性能的影响规律,并结合优化的工艺窗口,实现了最小线宽50 μm的多种图案微细电路的制造。制造的典型样件电导率为4.5×107 S/m,经100次附着力实验和100分钟超声实验后电阻变化率在1%左右;在电加热应用方面展现出优异的热响应速度,在3 V电压下最高温度可达158℃,能够在200 s内实现除冰。该技术为高效低成本制造纤维改性双马树脂复合材料基微细电路提供了一种有效方法,显示出良好的工业化应用前景。
-
关键词:
- 电场驱动微3D打印 /
- 石英纤维增强双马树脂基复合材料 /
- 非平整异质基材 /
- 增材制造 /
- 高精度电路
Abstract: Fiber-modified bismaleimide resin matrix composites are widely used in aerospace, smart skins, conformal antennas, electromagnetic shielding, high-frequency circuit substrates, and electrical heating by virtue of their excellent mechanical properties, high-temperature and corrosion-resistant characteristics. However, due to the non-flat, heterogeneous, and anisotropic characteristics of bismaleimide resin matrix composites, the simple, efficient, and low-cost fabrication of high-resolution circuits on this substrate is a current challenge to be solved. In this paper, a new method for fabricating high-precision circuits based on electric-field-driven micro-3D printing on quartz fiber modified bismaleimide resin matrix composites are proposed, and the basic forming principle and key technology implementation are described. Explored the characteristics of electric field distribution on the surface of non-flat heterogeneous composites and the changing law of field strength, and proposed a strategy to realize stable printing adjusting the threshold of electric field strength. The effects of the main process parameters on the precision, morphology and performance of the fabricated circuits were revealed experimentally, and the fabrication of various patterned circuits with a minimum line width of 50 μm had been realized by combining with an optimized process parameter. The typical sample manufactured had a conductivity of 4.5×107 S/m, and the resistance change rate was around 1% after 100 times of adhesion testing and 100 min ultrasonic experiments. It had excellent thermal response speed when applied to electric heating applications, and the maximum temperature can reach 158℃ under 3 V, and de-icing can be realized within 200s. This technology provides an effective method for the efficient and low-cost fabrication of fiber modified bismaleimide resin composite-based circuits, showing good prospects for industrial applications. -
表 1 主要实验设备信息
Table 1. Main experimental equipment information
Name Model number Factory owners Electric field-driven micro-3D printer EM3DP-4 A Self-research High temperature atmosphere furnaces GR.AF12/11 Shanghai guier machinery equipment co Vacuum drying oven DZF-6092 Shanghai yiheng scientific instrument co. Resistance tester ATL56 Suzhou penglian electronic equipment co. Infrared thermal imager NDJ-5 S Guangzhou ruimei electronic technology co. Constant voltage and constant current DC power supplies 85-2 Changzhou yinergy experimental instrument factory Ultrasonic cleaner SCQ—180323 P Shanghai shengyan ultrasonic co. -
[1] GAINA V, GAINA C. New Bismaleimide-Epoxy Resin System[J]. Polymer-Plastics Technology and Engineering, 2009, 48(5): 525-529. doi: 10.1080/03602550902824325 [2] CHEN W-C, CHEN Z-Y, BA Y, et al. Double-Decker-Shaped Polyhedral Silsesquioxanes Reinforced Epoxy/Bismaleimide Hybrids Featuring High Thermal Stability[J]. Polymers, 2022, 14(12): 2380. doi: 10.3390/polym14122380 [3] LI H, ZHAO L, QIAO Y, et al. Surface Treatment of Composites with Bismaleimide Resin-Based Wet Peel Ply for Enhanced Adhesive Bonding Performance[J]. Polymers, 2021, 13(20): 3488. doi: 10.3390/polym13203488 [4] XU J, CHEN P, MA S, et al. Synthesis, polymerization kinetics, and thermal properties of novel bismaleimides containing twisted structure[J]. Journal of Applied Polymer Science, 2023, 140(42): e54547. doi: 10.1002/app.54547 [5] YU L, YU Y, SHI J, et al. Synthesis of a Novel Hyperbranched Polyimide for Reinforcing Toughness and Insulating Properties of Bismaleimide Resin[J]. Polymers, 2022, 14(19): 4234. doi: 10.3390/polym14194234 [6] WU D, CHENG X, CHEN Z, et al. A flexible tactile sensor that uses polyimide/graphene oxide nanofiber as dielectric membrane for vertical and lateral force detection[J]. Nanotechnology, 2022, 33(40): 405205. doi: 10.1088/1361-6528/ac73a4 [7] HONG T, GUO S, JIANG W, et al. Highly Selective Frequency Selective Surface With Ultrawideband Rejection[J]. IEEE Transactions on Antennas and Propagation, 2022, 70(5): 3459-3468. doi: 10.1109/TAP.2021.3137453 [8] SHAO Y, WEI L, WU X, et al. Room-temperature high-precision printing of flexible wireless electronics based on MXene inks[J]. Nature Communications, 2022, 13(1): 3223. doi: 10.1038/s41467-022-30648-2 [9] DI RENZO M, ZAPPONE A, DEBBAH M, et al. Smart Radio Environments Empowered by Reconfigurable Intelligent Surfaces: How It Works, State of Research, and The Road Ahead[J]. IEEE Journal on Selected Areas in Communications, 2020, 38(11): 2450-2525. doi: 10.1109/JSAC.2020.3007211 [10] ZHAO Z, ZHU Y, WANG Z, et al. A biaxial stretchable, flexible electric heating composite film for de-icing[J]. Composites Part A:Applied Science and Manufacturing, 2022, 162: 107124. doi: 10.1016/j.compositesa.2022.107124 [11] SHAHAPURKAR K, GELAW M, TIRTH V, et al. Comprehensive review on polymer composites as electromagnetic interference shielding materials[J]. Polymers and Polymer Composites, 2022, 30: 096739112211021. [12] SHENG A, YU J, REN S. Printing Nanostructured Copper for Electromagnetic Interference Shielding[J]. ACS Applied Electronic Materials, 2022, 4(4): 2047-2052. doi: 10.1021/acsaelm.2c00199 [13] STEFANO J S, KALINKE C, DA ROCHA R G, et al. Electrochemical (Bio)Sensors Enabled by Fused Deposition Modeling-Based 3D Printing: A Guide to Selecting Designs, Printing Parameters, and Post-Treatment Protocols[J]. Analytical Chemistry, 2022, 94(17): 6417-6429. doi: 10.1021/acs.analchem.1c05523 [14] HYUN W J, SECOR E B, HERSAM M C, et al. High-Resolution Patterning of Graphene by Screen Printing with a Silicon Stencil for Highly Flexible Printed Electronics[J]. Advanced Materials, 2015, 27(1): 109-115. doi: 10.1002/adma.201404133 [15] CHEN C, RAN C, YAO Q, et al. Screen-Printing Technology for Scale Manufacturing of Perovskite Solar Cells[J]. Advanced Science, 2023, 10(28): 2303992. doi: 10.1002/advs.202303992 [16] CHEN S, FU H, SI Y, et al. Size effect enabling additive-free MXene ink with ultrahigh conductivity for screen printing of wireless electronics[J]. Nano Research, 2023, 16(8): 11012-11017. doi: 10.1007/s12274-023-5762-3 [17] LOHSE D. Fundamental Fluid Dynamics Challenges in Inkjet Printing[J]. Annual Review of Fluid Mechanics, 2022, 54(1): 349-382. doi: 10.1146/annurev-fluid-022321-114001 [18] LI X, LIU B, PEI B, et al. Inkjet Bioprinting of Biomaterials[J]. Chemical Reviews, 2020, 120(19): 10793-10833. doi: 10.1021/acs.chemrev.0c00008 [19] CRATON M T, ALBRECHT J D, CHAHAL P, et al. Multimaterial Aerosol Jet Printed Magnetic Nanocomposites for Microwave Circuits[J]. IEEE Transactions on Components, Packaging and Manufacturing Technology, 2021, 11(5): 865-871. doi: 10.1109/TCPMT.2021.3071113 [20] RAMESH S, MAHAJAN C, GERDES S, et al. Numerical and experimental investigation of aerosol jet printing[J]. Additive Manufacturing, 2022, 59: 103090. doi: 10.1016/j.addma.2022.103090 [21] WANG H, WANG J, CHEN D, et al. Robust Tattoo Electrode Prepared by Paper-Assisted Water Transfer Printing for Wearable Health Monitoring[J]. IEEE Sensors Journal, 2022, 22(5): 3817-3827. doi: 10.1109/JSEN.2022.3141457 [22] 兰红波, 李红珂, 钱垒, 等. 电场驱动喷射沉积微纳3D打印及其在先进电路和电子制造中的应用[J]. 机械工程学报, 2023, 59(9): 230-251.LAN Hongbo, LI Hongke, QIAN Lei, et al. Electric-field-driven Jet deposition micro-nano 3D printing and its applications in manufacturing advanced circuits and electronics[J]. Sci Sin Tech, 2023, 59(9): 230-251(in Chinese). [23] LI H, ZHANG Y, TAI Y, et al. Flexible transparent electromagnetic interference shielding films with silver mesh fabricated using electric-field-driven microscale 3D printing[J]. Optics & Laser Technology, 2022, 148: 107717. [24] SUN L, WANG R, ZHU X, et al. Low Cost and Facile Fabrication of a Micro-Mold with High Aspect Ratio for Transparent Electrodes with Metal Mesh Using Micro-Scale 3D Printing[J]. Advanced Materials Technologies, 2022, 7(12): 2200584. doi: 10.1002/admt.202200584 [25] ZHANG H, ZHU X, ZHOU J, et al. Microscale Hybrid Additive Manufacturing of Ultra-Fine, Embedded Cu/Ag(shell)–P4VP(core) Grid for Flexible Transparent Electrodes[J]. Advanced Materials Technologies, 2023, 8(8): 2201580. doi: 10.1002/admt.202201580 -
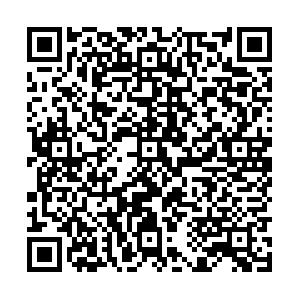
计量
- 文章访问数: 46
- HTML全文浏览量: 19
- 被引次数: 0