Research progress on testing methods, influencing factors and applications of graphene thermal conductivity
-
摘要: 石墨烯以其独特的结构和优异的导热性能引起研究者的广泛关注。石墨烯作为一种具有较高热导率的二维材料,在含能材料、电池材料、导热复合材料等领域都有重要应用。石墨烯导热的理论和实验研究有助于加强对固体导热机制的理解,可以为能源技术、电子器件热管理和散热技术的发展以及高效导热材料的设计提供参考。近年来,有较多关于石墨烯热导率的报道,对石墨烯热导率报道进行总结有利于相关研究人员更好地开展工作。本文对石墨烯热导率的测试方法、影响因素及应用现状进行了总结。首先介绍单层石墨烯、少层和多层石墨烯以及石墨烯基复合材料热导率测试方法,包括拉曼光谱法、热桥法、激光闪射法和3ω法。然后总结石墨烯热导率的理论研究成果,介绍石墨烯本征热导率的影响因素,如尺寸、层数和缺陷等对热导率的影响。随后归纳总结石墨烯导热材料在含能材料、电池材料和改性复合材料中的应用情况。最后,对石墨烯导热的研究进行了总结,提出目前石墨烯导热研究中的问题和挑战,并且对未来可能的发展方向做出展望。Abstract: Graphene has attracted much attention due to its unique structure and excellent thermal conductivity, which is widely used in energy materials, battery materials, thermal composite materials fields as a two-dimensional material with high thermal conductivity. It is of great significance to deepen the understanding of solid-state heat transfer mechanisms by theoretical and experimental research on the thermal conductivity of graphene, and it can guide the design of the development of energy technology, thermal management and heat dissipation technology of electronic devices and the high-efficiency thermal conductivity materials. In recent years, there are numerous reports on the thermal conductivity of graphene, and it is beneficial for researchers to carry out their work more effectively by summarizing these reports on the thermal conductivity of graphene. This work summarizes the testing methods, influencing factors, and application status of the thermal conductivity of graphene. Firstly, the thermal conductivity measurement methods of single-layer, few-layer and multilayer graphene and graphene matrix composites are introduced, including Raman spectroscopy, thermal bridge, laser flash and 3ω method. Then, the theoretical research achievements on the thermal conductivity of graphene was summarized and the influence factors of the intrinsic thermal conductivity of graphene such as size, number of layers and defects were discussed. Next, the application of graphene as a thermal conductor in energy materials, battery materials and modified composite materials is summarized. Finally, the research on graphene’s thermal conductivity is concluded and the current challenges and issues was highlighted in graphene’s thermal conductivity research. Moreover, the prospect for future development direction is proposed.
-
Key words:
- Graphene /
- Thermal conductivity /
- Raman method /
- Thermal bridge method /
- Phonon /
- Application
-
图 7 (a,b)悬浮和支撑石墨烯样品的悬浮微电热系统(METS) 扫描电镜图像; 比例尺: 5 μm。(c)悬浮样品S4的SEM图像;比例尺:1 μm。(d)装置的等效热电路,RSiNx是支撑样品的氮化物平台热阻[31]
Figure 7. (a, b) SEM images of the METS for suspended and supported graphene samples; scale bar: 5 µm. (c) SEM image of suspended sample S4; scale bar: 1 µm. (d) The equivalent thermal circuit of the device, RSiNx is the thermal resistance of the nitride platform for supported samples[31]
图 11 悬浮窄石墨烯 (Ly = 10 nm) 、方形石墨烯 (Lx = Ly) 和无限宽石墨烯的导热系数随石墨烯尺寸Lx的变化 (Ly =无穷) 。(a) 使用优化Tersoff势的结果和 (b) 使用反应经验键序 (REBO) 势的结果[59]
Figure 11. Thermal conductivity as functions of graphene size Lx for suspended narrow graphene (Ly = 10 nm) , square graphene (Lx = Ly) , and infifinite wide graphene (Ly = infinite) . (a) The result using optimized Tersoffff potential and (b) the result using Reactive Empirical Bond Order (REBO) potential[59]
表 1 部分石墨烯热导率测试方法及测试结果
Table 1. Test methods and results of thermal conductivity of some graphene
Sample Thermal conductivity/ (W·m−1·K−1) Measurement method Preparation method Reference Suspended Single-layer graphene 5300 ± 480 Raman Mechanical exfoliation 12 Suspended Single-layer graphene film 1224 ± 387 Raman and finite-element calculations Chemical vapor deposition(CVD) 16 Suspended single-layer graphene 1800 (325 K)
710 (500 K)Raman Mechanical exfoliation 17 Suspended single-layer graphene 600 Thermal bridge method Mechanical exfoliation 29 Supported 4 layers of graphene 1100 Comprehensive Raman CVD 30 Supported single-layer graphene 840 Comprehensive Raman CVD 30 Suspended single-layer graphene 850 Comprehensive Raman CVD 30 Suspended 2-layer graphene 970 Comprehensive Raman Mechanical exfoliation 30 Supported single-layer graphene 1100 Comprehensive Raman Mechanical exfoliation 30 Supported few-layer graphene 1250 Thermal bridge configuration Mechanical exfoliation 31 Few-layer graphene 176 I-V curve method CVD 32 -
[1] YU A, RAMESH P, SUN X, BEKYAROVA E, et al. Enhanced Thermal Conductivity in a Hybrid Graphite Nanoplatelet-Carbon Nanotube Filler for Epoxy Composites[J]. Advanced Materials, 2008, 20(24): 4740-4744. doi: 10.1002/adma.200800401 [2] KIM H S, JANG J U, LEE H, et al. Thermal Management in Polymer Composites: A Review of Physical and Structural Parameters[J]. Advanced Engineering Materials, 2018, 20(10): 1800204. doi: 10.1002/adem.201800204 [3] MADURANI K A, SUPRAPTO S, MACHRITA N I, et al. Progress in Graphene Synthesis and its Application: History, Challenge and the Future Outlook for Research and Industry[J]. ECS Journal of Solid State Science and Technology, 2020, 9(9): 093013. doi: 10.1149/2162-8777/abbb6f [4] STANKOVICH S, DIKIN D A, DOMMETT G H B, et al. Graphene-based composite materials[J]. Nature, 2006, 442(7100): 282-286. doi: 10.1038/nature04969 [5] SATTA T. Current Review on Synthesis, Composites and Multifunctional Properties of Graphene. [J] Topics in Current Chemistry, 2019, 377 (2): 10. [6] NOVOSELOV K S, FALl'KO V I, COLOMBO L, et al. A roadmap for graphene[J]. Nature, 2012, 490(7419): 192-200. doi: 10.1038/nature11458 [7] WEI Y J, YANG R G. Nanomechanics of graphene[J]. National Science Review, 2019, 6(2): 324-348. doi: 10.1093/nsr/nwy067 [8] 段淼, 李四中, 陈国华, 等. 机械法制备石墨烯的研究进展[J]. 材料工程, 2013, (12): 85-91.DUAN Miao, LI Sizhong, Chen G H, et al. Research progress in the preparation of graphene by mechanical method[J]. Materials Engineering, 2013, (12): 85-91(in Chinese). [9] 钱伟, 何大平, 李宝文, 等. 石墨烯基电磁屏蔽材料的研究进展[J]. 材料工程, 2020, 48 (07): 14-23.QIAN Wei, He Daping, Li Baowen, Research progress of graphene-based electromagnetic shielding materials[J]. Materials Engineering, 2020, 48 (07): 14-23(in Chinese). [10] 白明洁, 刘金龙, 齐志娜, 等. 石墨烯纳米流体研究进展[J]. 材料工程 2020, 48 (04): 46-59.BAI Mingjie, Liu Jinlong, Qi Zhina, et al. Research progress of graphene nanofluids[J]. Materials Engineering, 2020, 48 (04): 46-59(in Chinese). [11] CHEN Z, GAO P, LIU Z, et al. Graphene-Based LED: from Principle to Devices[J]. Acta Physico-Chimica Sinica, 2020, 36(1): 1907004. doi: 10.3866/PKU.WHXB201907004 [12] BALANDIN A A, GHOSH S, BAO W Z, CALIZO I, et al. Superior thermal conductivity of single-layer graphene[J]. Nano Letters, 2008, 8(3): 902-907. doi: 10.1021/nl0731872 [13] MOHAPATRA A, RAO M S R, JAISWAL M. Thermal transport in turbostratic multilayer graphene[J]. Carbon, 201 (2023): 120-128. [14] YANG X, HAN D, FAN H, et al. First-principles calculations of phonon behaviors in graphether: a comparative study with graphene[J]. Physical Chemistry Chemical Physics, 2021, 23(1): 123-130. doi: 10.1039/D0CP03191G [15] LEE M B, LEE C T, CHONG W W F, et al. Post-thermal annealed monolayer graphene healing elucidated by Raman spectroscopy[J]. Journal of Materials Science, 2023, 58(25): 10288-10302. doi: 10.1007/s10853-023-08685-z [16] BRAUN O, FURRER R, BUTTI P, et al. Spatially mapping thermal transport in graphene by an opto-thermal method[J]. npj 2D Materials and Applications 2022, 6 (1): 1-7. [17] LEE J U, YOON D, KIM H, et al. Thermal conductivity of suspended pristine graphene measured by Raman spectroscopy[J]. Physical Review B 2011, 83 (8): 081419. [18] BAJPAI A, SHARMA N, DWIVEDI P K, et al. Rapid Fabrication of Graphene Layers Over Polymeric Substrates Using Atmospheric Pressure Plasma Jet[J]. IEEE Transactions on Plasma Science 2023, 51 (3): 726-732. [19] M K R, BALAKRISHNAN J. Investigating the thermal transport in gold decorated graphene by opto-thermal Raman technique[J]. Nanotechnology 2022, 33 (13): 135706. [20] TARANNUM F, MUTHAIAH R, DANAYAT S, et al. Chemically Edge-Carboxylated Graphene Enhances the Thermal Conductivity of Polyetherimide–Graphene Nanocomposites[J]. ACS Applied Materials & Interfaces, 2022, 14(12): 14753-14763. [21] DONG Z. Y, Zhou Y, CHEN X. Q, et al. Effect of low-frequency optical phonons on the thermal conductivity of 2H molybdenum disulfide[J]. Physical Review B, 2022, 105(18): 184301. doi: 10.1103/PhysRevB.105.184301 [22] WANG N, YANG Z, WANG Y, et al. Interface and properties of inorganic fullerene tungsten sulphide nanoparticle reinforced poly (ether ether ketone) nanocomposites[J]. Results in Physics, 2017, 7: 2417-2424. doi: 10.1016/j.rinp.2017.07.018 [23] FENG Y, SATO Y, INOUE T, et al. Enhanced Thermal Conductivity of Single-Walled Carbon Nanotube with Axial Tensile Strain Enabled by Boron Nitride Nanotube Anchoring[J]. Small, 2023, 2308571: 1-8. [24] BONERA E. MOLLE A. Optothermal Raman Spectroscopy of Black Phosphorus on a Gold Substrate[J]. Nanomaterials, 2022, 12(9): 1410. doi: 10.3390/nano12091410 [25] ALI F, AHMED F, YANG Z, et al. Energy Dissipation in Black Phosphorus Heterostructured Devices[J]. Advanced Materials Interfaces, 2019, 6(2): 1801528. doi: 10.1002/admi.201801528 [26] HONE J, WHITNEY M, ZETTL A. Thermal conductivity of single-walled carbon nanotubes[J]. Synthetic Metals, 1999, 103(1): 2498-2499 [27] POP E, VARSHNEY V, ROY A K, Thermal properties of graphene: Fundamentals and applications[J]. Mrs Bulletin, 2012, 37 (12): 1273-1281. [28] HU S, XU B, ZHAO Y, et al. Preparation of CNTs/PP@Gr composites with a segregated structure and enhanced electrical and thermal conductive properties by the Pickering emulsion method[J]. Composites Science and Technology, 2022, 222: 109374. doi: 10.1016/j.compscitech.2022.109374 [29] SEOL J H, JO I, MOORE A L, et al. Two-Dimensional Phonon Transport in Supported Graphene[J]. Science, 2010, 328(5975): 213-216. doi: 10.1126/science.1184014 [30] LI Q Y, XIA K, ZHANG J, et al. Measurement of specific heat and thermal conductivity of supported and suspended graphene by a comprehensive Raman optothermal method[J]. Nanoscale, 2017, 9(30): 10784-10793. doi: 10.1039/C7NR01695F [31] WANG Z, XIE R, BUI C T, et al. Thermal Transport in Suspended and Supported Few-Layer Graphene[J]. Nano Letters, 2011, 11(1): 113-118. doi: 10.1021/nl102923q [32] MUNOZ E, LU J X, YAKOBSON B I. Ballistic Thermal Conductance of Graphene Ribbons[J]. Nano Letters, 2010, 10(5): 1652-1656. doi: 10.1021/nl904206d [33] PETTES M T, JI H, RUOFF R S, et al. Thermal transport in three-dimensional foam architectures of few-layer graphene and ultrathin graphite[J]. Nano Letters, 2012, 12(6): 2959-64. doi: 10.1021/nl300662q [34] 金玲, 李红侠, 倪俊. 激光闪射法对石墨烯薄膜导热性能的研究[J]. 能源研究与利用2020, (01): 46-48.JIN Ling, LI Hongxia, NI Jun. Study on thermal conductivity of graphene thin films by laser flash method[J]. Energy Research And Utilization, 2020, (01): 46-48 (in Chinese). [35] 张振威, 蒋锐, 朱宇瑾, 等. 基于激光闪射法测量Zr基非晶合金的导热系数[J]. 中国材料进展, 2023, 42(2): 181-184.ZHANG Zhenwei, JIANG Rui, ZHU Yujing, et al. Measurement of thermal conductivity of Zr-based amorphous alloys by laser flash method[J]. Progress Of Materials In China, 2023, 42(2): 181-184 (in Chinese). [36] LI C, LIU Z, ZHANG X, et al. Thermal transport measurement of three-dimensional graphene powders for application in energy devices[J]. Materials Today Energy, 2021, 19: 100582. doi: 10.1016/j.mtener.2020.100582 [37] GóMEZ-GóMEZ A, RAMíREZ C, LLORENTE J, et al. Improved crack resistance and thermal conductivity of cubic zirconia containing graphene nanoplatelets[J]. Journal of the European Ceramic Society, 2020, 40(4): 1557-1565. doi: 10.1016/j.jeurceramsoc.2019.12.016 [38] ZHANG Z, GKARTZOU E, JESTIN S, et al. 3D Printing Processability of a Thermally Conductive Compound Based on Carbon Nanofiller-Modified Thermoplastic Polyamide 12[J]. Polymers-Basel, 2022, 14(470): 1-16. [39] SADEGH MOGHANLOU F, VAJDI M, JAFARZADE H, et al. Spark plasma sinterability and thermal diffusivity of TiN ceramics with graphene additive[J]. Ceramics International, 2021, 47(7): 10057-10062. doi: 10.1016/j.ceramint.2020.12.152 [40] VAJDI M, SADEGH MOGHANLOU F, NEKAHI S, et al. Role of graphene nano-platelets on thermal conductivity and microstructure of TiB2–SiC ceramics[J]. Ceramics International, 2020, 46(13): 21775-21783. doi: 10.1016/j.ceramint.2020.05.289 [41] 刘黎, 周旺枝, 张洪雷. 激光法测量材料热物理性能的原理及方法[J]. 武钢技术, 2013, 51(3): 9-12.LI NIU, ZHI WANGZHOU, HOULEI ZHANG. Test principle and measuring procedure for thermo physical properties of materials by laser flash method[J]. Wisco Technology, 2013, 51(3): 9-12 (in Chinese). [42] 何龙. 3ω法在薄膜界面热阻测量中的应用 [D]. 成都: 电子科技大学, 2016.LONG HE. Application of the 3-omega method on measuring the interfacial thermal resistance in thin films [D]. Chengdu: University of Electronic Science and Technology of China, 2016(in Chinese). [43] CAHILL D G, POHLR O. Thermal conductivity of amorphous solds above the plateau[J]. Physical Review B Condensed Matter, 1987, 35(8): 4067-4073. doi: 10.1103/PhysRevB.35.4067 [44] CAHILLD G. Thermal conductivity measurement from 30 to 750 K: the 30 method [J] Review of Scientific Instruments, 1990, 61 (2): 802-808. [45] CAHILL D G, Katiyar M, Abelson JR. Thermal conductivity of a-Si: H thin films[J]. Physical review B, 1994, 50(9): 6077-6081. doi: 10.1103/PhysRevB.50.6077 [46] 吴熔琳, 邵铮铮, 石剑豪, 等. 3ω方法测量微纳米材料热物性研究进展[J]. 材料导报, 2013, 27(S2): 91-94.WU RONGLIN, SHAO ZHENGZHENG, SHI JIANHAO, et al. Research Progress on the Measurement of the Thermal Physical Property of Micro/Nano Materials by 3ω Method[J]. Material guide, 2013, 27(S2): 91-94(in Chinese). [47] ZHANG Y, EDWARDS M, SAMANI M K, et al. Characterization and simulation of liquid phase exfoliated graphene-based films for heat spreading applications[J]. Carbon, 2016, 106: 195-201. doi: 10.1016/j.carbon.2016.05.014 [48] JAGANNADHAM K. Thermal conductivity of copper-graphene composite films synthesized by electrochemical deposition with exfoliated graphene platelets[J]. Metallurgical and Materials Transactions B, 2012, 43B: 316-324. [49] QIU-HAO ZHU, JING-SONG PENG, XIAO GUO, et al. Accurate determination of anisotropic thermal conductivity for ultrathin composite film[J]. Chinese Physics B, 2022, 31: 108102. doi: 10.1088/1674-1056/ac6ee5 [50] KNURAG A, AYYAGARI N; FISHER T S. Effects of Graphene Nanopetal Outgrowths on Internal Thermal Interface Resistance in Composites[J]. Acs Appl Mater Inter, 2016, 8(10): 6678-6684. doi: 10.1021/acsami.5b11796 [51] Karthik R, Nagarjann R H, Raja B, et al. Thermal conductivity of CuO-DI water nanofluids using 3o measurement technique in a suspended microwire[J]. Experimental Thermmal Fluid Science, 2012, 40: 1-9. doi: 10.1016/j.expthermflusci.2012.01.006 [52] 唐大伟, 王照亮. 微纳米材料和结构热物理特性表征 [M]. 北京: 科学出版社, 2010 : 5.TANG DAWEI, WANG ZHAOLIANG. Thermophysical characterization of micro and nano materials and structures [M]. Beijing: Science Press, 2010: 5. [53] YANG Z, WANG R, LI H, et al. Phonon thermal transport in polycrystalline graphene: Effects of grain, vacancy and strain[J]. International Journal of Heat and Mass Transfer, 2023, 209: 124057. doi: 10.1016/j.ijheatmasstransfer.2023.124057 [54] CHEN J, ZHANG G, LI B W. Substrate coupling suppresses size dependence of thermal conductivity in supported graphene[J]. Nanoscale, 2013, 5(2): 532-536. doi: 10.1039/C2NR32949B [55] THOMAS J A, IUTZI R M, MCGAUGHEY A J H. Thermal conductivity and phonon transport in empty and water-filled carbon nanotubes[J]. Physical Review B, 2010, 81(4): 045413. doi: 10.1103/PhysRevB.81.045413 [56] WANG H D, KURATA K, FUKUNAGA T, et al. Width-dependent intrinsic thermal conductivity of suspended monolayer graphene[J]. International Journal of Heat and Mass Transfer, 2017, 105: 76-80. doi: 10.1016/j.ijheatmasstransfer.2016.09.054 [57] NIKA D L, GHOSH S, POKATILOV E P, et al. Lattice thermal conductivity of graphene flakes: Comparison with bulk graphite[J]. Applied Physics Letters, 2009, 94(20): 203103. doi: 10.1063/1.3136860 [58] CHEN J, LIU B. Dimension-dependent thermal conductivity of graphene nanoribbons on silicon carbide[J]. The European Physical Journal Plus, 2021, 136(4): 379. doi: 10.1140/epjp/s13360-021-01386-z [59] SU R X, ZHANG X. Size effect of thermal conductivity in monolayer graphene[J]. Applied Thermal Engineering, 2018, 144: 488-494. doi: 10.1016/j.applthermaleng.2018.08.062 [60] BAO W, WANG Z, CHEN G. Substrate effect and temperature dependence of thermal transport characteristics in GaN-based graphene[J]. International Journal of Heat and Mass Transfer, 2021, 173: 121266. doi: 10.1016/j.ijheatmasstransfer.2021.121266 [61] BALANDIN A A. Thermal properties of graphene and nanostructured carbon materials[J]. Nature Materials, 2011, 10(8): 569-81. doi: 10.1038/nmat3064 [62] NIKA D L, POKATILOV E P, ASKEROV A S, et al. Phonon thermal conduction in graphene: Role of Umklapp and edge roughness scattering[J]. Physical Review B, 2009, 79(15): 155413. doi: 10.1103/PhysRevB.79.155413 [63] LINDSAY L, Li W, CARRETE J, et al. Phonon thermal transport in strained and unstrained graphene from first principles[J]. Physical Review B, 2014, 89(15): 155426. doi: 10.1103/PhysRevB.89.155426 [64] FENG T, RUAN X, YE Z, et al. Spectral phonon mean free path and thermal conductivity accumulation in defected graphene: The effects of defect type and concentration[J]. Physical Review B, 2015, 91(22): 224301. doi: 10.1103/PhysRevB.91.224301 [65] MINNICH A J, JOHNSON J A, SCHMIDT A J, et al. Thermal Conductivity Spectroscopy Technique to Measure Phonon Mean Free Paths[J]. Physical Review Letters, 2011, 107(9): 095901. doi: 10.1103/PhysRevLett.107.095901 [66] MINNICH A J. Determining phonon mean free paths from observations of quasiballistic thermal transport[J]. Physical Review Letters, 2012, 109(20): 205901. doi: 10.1103/PhysRevLett.109.205901 [67] HAO F, FANG D, XU Z. Mechanical and thermal transport properties of graphene with defects[J]. Applied Physics Letters, 2011, 99(4): 041901. doi: 10.1063/1.3615290 [68] MALEKPOUR H, RAMNANI P, SRINIVASAN S, et al. Thermal conductivity of graphene with defects induced by electron beam irradiation[J]. Nanoscale, 2016, 8(30): 14608-16. doi: 10.1039/C6NR03470E [69] ZHAO W, WANG Y, WU Z, et al. Defect-Engineered Heat Transport in Graphene: A Route to High Efficient Thermal Rectification[J]. Scientific Reports, 2015, 5: 11962. doi: 10.1038/srep11962 [70] KIM K S, ZHAO Y, JANG H, et al. Large-scale pattern growth of graphene films for stretchable transparent electrodes[J]. Nature, 2009, 457(7230): 706-710. doi: 10.1038/nature07719 [71] HOFMANN M, NEZICH D, REINA A, et al. In-Situ Sample Rotation as a Tool to Understand Chemical Vapor Deposition Growth of Long Aligned Carbon Nanotubes[J]. Nano Letters 2008, 8 (12): 4122-4127. [72] LI X S, CAI W W, AN J H, et al. Large-Area Synthesis of High-Quality and Uniform Graphene Films on Copper Foils[J]. Science 2009, 324 (5932): 1312-1314. [73] LEE W, KIHM K D, KIM H G, et al. In-plane thermal Conductivity of Polycrystalline Chemical Vapor Deposition Graphene with Controlled Grain Sizes[J]. Nano Letters, 2017, 17(4): 2361-2366. doi: 10.1021/acs.nanolett.6b05269 [74] LEE D, LEE S, AN B S, et al. Dependence of the In-Plane Thermal Conductivity of Graphene on Grain Misorientation[J]. Chemistry of Materials, 2017, 29(24): 10409-10417. doi: 10.1021/acs.chemmater.7b03821 [75] SEROV A Y, ONG Z Y, POP E. Effect of grain boundaries on thermal transport in graphene[J]. Applied Physics Letters, 2013, 102(3): 033104. doi: 10.1063/1.4776667 [76] AKSAMIJA Z, KNEZEVIC I. Lattice thermal transport in large-area polycrystalline graphene[J]. Physical Review B, 2014, 90(3): 035419. doi: 10.1103/PhysRevB.90.035419 [77] YAZYEV O V, LOUIE S G. Topological defects in graphene: Dislocations and grain boundaries[J]. Physical Review B, 2010, 81(19): 195420. doi: 10.1103/PhysRevB.81.195420 [78] BAGRI A, KIM S P, RUOFF R S, et al. Thermal transport across twin grain boundaries in polycrystalline graphene from nonequilibrium molecular dynamics simulations[J]. Nano Letters, 2011, 11(9): 3917-3921. doi: 10.1021/nl202118d [79] MORTAZAVI B, POTSCHKE M, CUNIBERTI G. Multiscale modeling of thermal conductivity of polycrystalline graphene sheets[J]. Nanoscale 2014, 6 (6): 3344-3352. [80] LAI S, KYU JANG S, JAE SONG Y, et al. Probing graphene defects and estimating graphene quality with optical microscopy[J]. Applied Physics Letters, 2014, 104(4): 043101. doi: 10.1063/1.4863080 [81] MA T, LIU Z, WEN J, et al. Tailoring the thermal and electrical transport properties of graphene films by grain size engineering[J]. Nature Communications, 2017, 8: 14486. doi: 10.1038/ncomms14486 [82] YASAEI P, FATHIZADEH A, HANTEHZADEH R, et al. Bimodal Phonon Scattering in Graphene Grain Boundaries[J]. Nano Letterss, 2015, 15(7): 4532-4540. doi: 10.1021/acs.nanolett.5b01100 [83] ADETAYO A E, AHMED T N, ZAKHIDOV A, et al. Improvements of Organic Light-Emitting Diodes Using Graphene as an Emerging and Efficient Transparent Conducting Electrode Material[J]. Advanced Optical Materials, 2021, 9(14): 2002102. doi: 10.1002/adom.202002102 [84] CHEGEL R. Magneto-electronic and thermopower properties of B, N and Si doped monolayer graphene[J]. Diamond and Related Materials, 2023, 137: 110154. doi: 10.1016/j.diamond.2023.110154 [85] HU SHIQIAN, CHEN JIE, YANG NUO, et al. Thermal Transport in Graphene with Defect and Doping: PhononModes Analysis[J]. Carbon, 2017, 116: 139-144 doi: 10.1016/j.carbon.2017.01.089 [86] CHIEN S K, YANG Y T, CHEN C O K. Influence of Chemisorption on the Thermal Conductivity of GrapheneNanoribbons[J]. Carbon, 2012, 50(2): 421-428. doi: 10.1016/j.carbon.2011.08.056 [87] 斯超, 陆规, 王晓东, 等. 不同类型缺陷对多层石墨烯热导率的影响[J]. 工程热物理学报, 2018, 39(9): 2012-2017.SI CHAO, LU GUI, WANG XIAO-DONG, et al. The Effect of Defect Type on the Thermal Conductivity of Multi-layer Graphene[J]. Journal of Engineering Thermophysics, 2018, 39(9): 2012-2017(in Chinese). [88] Yang B, Li D, Yang H, et al. Thermal conductivity enhancement of defective graphene nanoribbons[J]. International Communications in Heat and Mass Transfer, 2020, 117: 104735. doi: 10.1016/j.icheatmasstransfer.2020.104735 [89] CHEGEL R. Magneto-electronic and thermopower properties of B, N and Si doped monolayer graphene[J]. Diamond and Related Materials, 2023, 137: 110154. doi: 10.1016/j.diamond.2023.110154 [90] CHEN S, WU Q, MISHRA C, et al. Thermal conductivity of isotopically modified graphene[J]. Nature Materials, 2012, 11(3): 203-207. doi: 10.1038/nmat3207 [91] SIKDER A K, SIKDER N. A review of advanced high-performance, insensitive and thermally stable energetic materials emerging for military and space applications[J]. Journal of Hazardous Materials, 2004, 112(1-2): 1-15. doi: 10.1016/j.jhazmat.2004.04.003 [92] M B TALAWARA, R SIVABALAN, M ANNIYAPPEN, et al. Emerging Trends in Advanced High Energy Materials[J]. Combustion Explosion and Shock Waves, 2007, 43(1): 62-72. doi: 10.1007/s10573-007-0010-9 [93] SINGH H, SOMAYAJULU M R, RAO R B, et al. Influence of carbon on combustion characteristics of Magnesium-Sodium Nitrate Propellant[J]. Propellants, Explosives, Pyrotechnics, 1991, 16(3): 115-118. doi: 10.1002/prep.19910160305 [94] LI R, WANG J, SHEN J P, et al. Preparation and Characterization of Insensitive HMX/Graphene Oxide Composites[J]. Propellants, Explosives, Pyrotechnics, 2013, 38(6): 798-804. doi: 10.1002/prep.201200199 [95] REN S, RONG P, YU Q, et al. Preparations, properties and applications of graphene in functional devices: A concise review[J]. Ceramics International, 2018, 44(11): 11940-11955. doi: 10.1016/j.ceramint.2018.04.089 [96] SABOURIN J L, DABBS D M, YETTER R A, et al. Functionalized Graphene Sheet Colloids for Enhanced Fuel/Propellant Combustion[J]. ACS Nano, 2009, 3(12): 3945-3954. doi: 10.1021/nn901006w [97] STANKOVICH S, DIKIN D A, Dommett G H, et al. Graphene-based composite materials[J]. Nature, 2006, 442(7100): 282-286. doi: 10.1038/nature04969 [98] BERMAN D, ERDEMIR A, SUMANT A V. Graphene: a new emerging lubricant[J]. Materials Today, 2014, 17(1): 31-42. doi: 10.1016/j.mattod.2013.12.003 [99] DENG P, REN H, JIAO Q. Enhanced the combustion performances of ammonium perchlorate-based energetic molecular perovskite using functionalized graphene[J]. Vacuum, 2019, 169: 108882. doi: 10.1016/j.vacuum.2019.108882 [100] ZHANG M, ZHAO F, LI H, et al. Insight into graphene-salen metal nanocomposites on combustion performance and mechanism of HMX-CMDB propellant[J]. Chemical Engineering Journal, 2022, 429: 132175. doi: 10.1016/j.cej.2021.132175 [101] JAIN S, PARK W, CHEN Y P, et al. Flame speed enhancement of a nitrocellulose monopropellant using graphene microstructures[J]. Journal of Applied Physics, 2016, 120(17): 174902. doi: 10.1063/1.4966933 [102] 林聪妹, 刘佳辉, 何冠松, 等. 石墨对TATB基PBX导热性能的影响[J]. 含能材料, 2015, 23(11): 1119-1123.LIN Congmei, LIU Jiahui, He Guansong, et al. Effect of graphite on thermal conductivity of TATB based PBX[J]. Energetic Materials, 2015, 23(11): 1119-1123(in Chinese). [103] 张建虎, 潘丽萍, 丁玲, 等. 溶剂悬浮法制备含铝炸药造型粉及其性能. 兵器装备工程学报[J]. 2021, 42 (06): 282-286.ZHANG J H, PAN L P, DING L, et al. Preparation of aluminum-containing explosive molding powder by solvent suspension method and its properties[J]. Journal of Ordnance Equipment Engineering, 2021, 42 (06): 282-286(in Chinese). [104] 徐露萍, 李念念, 郭惠丽, 等. 国外PBX炸药装药安全贮存寿命评价研究[J]. 兵器装备工程学报, 2020, 41(7): 164-168.XU Luping, LI Nian-Nian, GUO Hui-Li, et al. Study on safe storage life evaluation of foreign PBX explosive charge[J]. Journal of Ordnance Equipment Engineering, 2020, 41(7): 164-168(in Chinese). [105] LIU Y, HUL S, GONG S, et al. Study of ammonium perch lo-rate-based molecular perovskite (H, DABCO) [NH(CIO)]/graphene energetic composite with insensitive performance[J]. Central European Journal of Energetic Materials, 2020, 17(3): 451-469. doi: 10.22211/cejem/127934 [106] LI R, WANG J, SHEN J P, et al. Preparation and Characterization of Insensitive HMX/Graphene Oxide Composites[J]. Propellants, Explosives, Pyrotechnics, 2013, 38(6): 798-804. doi: 10.1002/prep.201200199 [107] WANG J, CHEN S, YAO Q, et al. Preparation, Characterization, Thermal Evaluation and Sensitivities of TKX-50/GO Composite[J]. Propellants, Explosives, Pyrotechnics, 2017, 42(9): 1104-1110. doi: 10.1002/prep.201700080 [108] XIANRUI S, CHUANGDONG L, Lu T, et al. Tuning the micro-structure, laser ignition, and isovolumetric burning performance of nitrocellulose propellant via incorporation of graphene oxide[J]. Polymer Composites, 2023, 44(6): 3245-3254. doi: 10.1002/pc.27315 [109] 吴凯. 石墨烯对RDX机械感度及爆轰性能的影响[J]. 中国安全生产科学技术, 2020, 16(3): 88-92.WU Kai. Influence of graphene on mechanical sensitivityand detonation performance of RDX[J]. Journal of Safety Science and Technology, 2020, 16(3): 88-92(in Chinese). [110] 潘丽萍, 张建虎, 何冠松, 等. 石墨烯微结构对TATB基复合炸药导热性能的影响[J]. 兵器装备工程学报 2022, 43 (09): 338-342.PAN Liping, ZHANG Jianhu, HE Guansong, et al. Effect of graphene microstructure on thermal conductivity of TATB-based composite explosive[J]. Journal of Ordnance Equipment Engineering, 2022, 43 (09): 338-342(in Chinses). [111] WILLEY T M, VAN BUUREN T, LEE J R I, et al. Changes in Pore Size Distribution upon Thermal Cycling of TATB-based Explosives Measured by Ultra-Small Angle X-Ray Scattering[J]. Propellants, Explosives, Pyrotechnics, 2006, 31(6): 466-471. doi: 10.1002/prep.200600063 [112] LIN C, NIE S, He G, et al. Construction of efficient thermally conductive networks with macroscopic separated architectures for polymer based energetic composites[J]. Composites Part B:Engineering, 2020, 203: 108447. doi: 10.1016/j.compositesb.2020.108447 [113] WANG L, LIU H. Performance studies of PEM fuel cells with interdigitated flow fields[J]. Journal of Power Sources, 2004, 134(2): 185-196. doi: 10.1016/j.jpowsour.2004.03.055 [114] ZHOU B, ZHANG X, ZHANG G, et al. Thermally conductive process and mechanism of TATB-based polymer-bonded explosives filled with graphene: Effects of interfacial thermal resistance and morphology/content of graphene[J]. Polymer Engineering & Science, 2023, 63(10): 3411-3419. [115] HE G, YANG Z, ZHOU X, et al. Polymer bonded explosives (PBXs) with reduced thermal stress and sensitivity by thermal conductivity enhancement with graphene nanoplatelets[J]. Composite Science and Technology, 2016, 131: 22-31. doi: 10.1016/j.compscitech.2016.05.009 [116] XIAO L Q, ZHAO Y, FU X L, et al. Mechanical Sensitivity Reduction of Energetic Materials by Using Carbon Nanomaterials[J]. Journal of Physics:Conference Series, 2023, 2478(3): 032010. doi: 10.1088/1742-6596/2478/3/032010 [117] LIU Y, LEHNERT W, JANBN H, et al. A review of high-temperature polymer electrolyte membrane fuel-cell (HT-PEMFC)-based auxiliary power units for diesel-powered road vehicles[J]. Journal of Power Sources, 2016, 311: 91-102. doi: 10.1016/j.jpowsour.2016.02.033 [118] HAN C, CHEN Z. Study on the synergism of thermal transport and electrochemical of PEMFC based on N, P co-doped graphene substrate electrode[J]. Energy, 2021, 214: 118808. doi: 10.1016/j.energy.2020.118808 [119] HAN C, JIANG T, SHANG K, et al. Heat and mass transfer performance of proton exchange membrane fuel cells with electrode of anisotropic thermal conductivity[J]. International Journal of Heat and Mass Transfer, 2022, 182: 121957. doi: 10.1016/j.ijheatmasstransfer.2021.121957 [120] 黄菊花, 陈强, 曹铭, 等. 石蜡/膨胀石墨/石墨烯/铝蜂窝复合相变材料的制备及锂电池控温性能研究[J]. 化工新型材料, 2022, 50(2): 140-144+149.HUANG Juhua, CHEN Qiang, CAO Ming, et al. Research on preparation of PW/EG/graphene/Al honeycomb CPCM and temperature control performcane of lithium battery[J]. New Chemical Materials, 2022, 50(2): 140-144+149(in Chinese). [121] XU Z, BAI J-Q, RAO C, et al. Facile Preparation of Silver-decorated Graphene in Polyvinylidene Fluoride for Highly Enhanced Thermal Conductivity[J]. Chemistryselect 2022, 7 (30): e202200827. [122] CHENG S, GUO X, TAN P, et al. A graphene aerogel with reversibly tunable thermal resistance for battery thermal management[J]. Journal of Materials Chemistry A, 2023, 11(33): 17779-17786 doi: 10.1039/D3TA03190J [123] ZHAO B, JIANG L, ZENG X, et al. A highly thermally conductive electrode for lithium ion batteries[J]. Journal of Materials Chemistry A, 2016, 4(38): 14595-14604. doi: 10.1039/C6TA04774B [124] LIN Y, KANG Q, WEI H, et al. Spider Web-Inspired Graphene Skeleton-Based High Thermal Conductivity Phase Change Nanocomposites for Battery Thermal Management[J]. Nano-Micro Letters, 2021, 13(1): 180. doi: 10.1007/s40820-021-00702-7 [125] BRINKWORTH B J, CROSS B M, MARSHALL R H, et al. Thermal regulation of photovoltaic cladding[J]. Solar Energy, 1997, 61(3): 169-178. doi: 10.1016/S0038-092X(97)00044-3 [126] 陈志能, 丁辉, 吴淑英, 等. 基于复合相变散热的锂离子电池热管理实验研究[J]. 化工新型材料, 2021, 49(2): 144-148.CHEN Zhneng, DING Hui, WU Shuying, et al. Experimental study on thermal management of Li-ion batteries based on composite phase change heat dissipation[J]. New Chemical Materials, 2021, 49(2): 144-148(in Chinese). [127] 赵静, 张建安, 吴明元, 等. 填充型导热聚合物基复合材料的研究进展[J]. 化工新型材料, 2017, 45(8): 3-5.ZHAO Jing, ZHANG Jianan, WU Mingyuan, et al. Research progress of filled thermal conductive polymer matrix composites[J]. New Chemical Materials, 2017, 45(8): 3-5(in Chinese). [128] WONG T T, LAU K T, TAM W Y, et al. Effects of silane surfactant on Nano-ZnO and rheology properties of nano-ZnO/epoxy on the UV absorbability of nano-ZnO/epoxy/micron-HGF composite[J]. Composites Part B:Engineering, 2016, 90: 378-385. doi: 10.1016/j.compositesb.2016.01.005 [129] ZHAO Y H, ZHANG Y F, WU Z K, et al. Synergic enhancement of thermal properties of polymer composites by graphene foam and carbon black[J]. Composites Part B:Engineering, 2016, 84: 52-58. doi: 10.1016/j.compositesb.2015.08.074 [130] CUI T, LI Q, Xuan Y, et al. Preparation and thermal properties of the graphene–polyolefin adhesive composites: Application in thermal interface materials[J]. Microelectronics Reliability, 2015, 55 (12, Part A): 2569-2574. [131] 欧阳泽宇, 王珂珂, 饶琼, 等. 石墨烯纳米片/(酚酞聚芳醚酮-环氧树脂) 双逾渗导热复合材料的制备和性能[J]. 复合材料学报, 2021, 38(3): 722-731.OUYANG ZEYU, WANG KEKE, RAO Qiong, et al. Preparation and properties of thermally conductive graphene nanoplates/(polyether ketone cardo-epoxy) composites with double percolation structures[J]. Acta Materiae Compositae Sinica, 2021, 38(3): 722-731(in Chinese). [132] WU B, LI Y, CHEN W, et al. A dual non-covalent bonding constructed continuous interfacial structure for reducing interfacial thermal resistance[J]. Journal of Materials Chemistry A, 2022, 10(26): 13858-13867. doi: 10.1039/D2TA02651A [133] CHU K, LI Ws, DONG H, et al. Role of graphene waviness on the thermal conductivity of graphene composites[J]. Applied Physics A, 2013, 111(1): 221-225. doi: 10.1007/s00339-012-7497-y [134] TANG B, HU G, GAO H, et al. Application of graphene as filler to improve thermal transport property of epoxy resin for thermal interface materials[J]. International Journal of Heat and Mass Transfer, 2015, 85: 420-429. doi: 10.1016/j.ijheatmasstransfer.2015.01.141 [135] 史智慧, 李莹, 闫璐, 等. 液相剥离法制备石墨烯及在导热硅橡胶中的应用[J]. 无机材料学报, 2017, 32(9): 955-960. doi: 10.15541/jim20160659SHI Zhihui, LI YING, Yan Lu, et al. Preparation of graphene by liquid phase stripping method and its application in thermal conductive silicone rubber[J]. Journal of Inorganic Materials, 2017, 32(9): 955-960(in Chinese). doi: 10.15541/jim20160659 [136] XIAOKUN GU, YUJIE WEI, XIAOBO Yin, et al. Colloquium: Phononic thermal properties of two-dimensional materials[J]. Reviews of modern physics, 2018, 90(4): 041002. doi: 10.1103/RevModPhys.90.041002 [137] VILLAROMAN D, WANG X, DAI W, et al. Interfacial thermal resistance across graphene/Al2O3 and graphene/metal interfaces and post-annealing effects[J]. Carbon, 2017, 123: 18-25. doi: 10.1016/j.carbon.2017.07.039 [138] LIU D K, ZHAO J X, NING Y Y, et al. Constructing zebraskin structured graphene/copper composites with ul-trahigh thermal conductivity[J]. Composites Communications, 2021, 25: 100704. doi: 10.1016/j.coco.2021.100704 [139] HONG Y, JU M G, ZHANG J, et al. Phonon thermal transport in a graphene/MoSe2 van der Waals heterobilayer[J]. Physical Chemistry Chemical Physics, 2018, 20(4): 2637-2645. doi: 10.1039/C7CP06874C [140] LIN S, BUEHLER M J. The effect of non-covalent functionalization on the thermal conductance of graphene/organic interfaces[J]. Nanotechnology, 2013, 24(16): 165702. doi: 10.1088/0957-4484/24/16/165702 [141] QIU L, GUO P, KONG Q, et al. Coating-boosted interfacial thermal transport for carbon nanotube array nano-thermal interface materials[J]. Carbon, 2019, 145: 725-733. doi: 10.1016/j.carbon.2019.01.085 [142] WANG M, GALPAYA D, LAI Z B, et al. Surface functionalization on the thermal conductivity of graphene–polymer nanocomposites[J]. International Journal of Smart and Nano Materials, 2014, 5(2): 123-132. doi: 10.1080/19475411.2014.904828 [143] 唐波. 石墨烯修饰的复合热界面材料的热性能研究[J]. 化学通报 2022, 85(12): 1483-1487.TANG Bo. Study on thermal properties of graphene-modified composite thermal interface materials[J]. Chinese Chemical Bulletin, 2022, 85(12): 1483-1487(in Chinese). [144] 邢伟义, 陈亮, 周慕天, 等. 氮化硼/石墨烯复合导热填料的制备及其环氧树脂复合材料阻燃导热绝缘性能的研究[J]. 中国科学: 化学 2023, 53 (02): 207-216.XING Weiyi, CHEN Liang, ZHOU Mutian, et al. Preparation of Boron nitride/graphene composite thermal conductive filler and Study on Flame retardant Thermal insulation Properties of Epoxy resin composite[J]. Science in China: Chemistry, 2023, 53 (02): 207-216(in Chinese). [145] 贾锡宁, 王严, 石慧, 等. 兼具阻燃和导热性能的环氧树脂复合材料: 石墨烯纳米片杂化三聚氰胺磷酸盐的作用[J]. 复合材料学报, 2023, 40(3): 1395-1405JIA Xi'ning, WANG Yan, SHI Hui, et al. Epoxy resin composites with flame retardancy and thermal conductivity: Effect of graphene nanoplatelets hybridized with melamine phosphate[J]. Acta Materiae Compositae Sinica, 2023, 40(3): 1395-1405(in Chinese). [146] GUO Y, YANG X, RUAN K, et al. Reduced Graphene Oxide Heterostructured Silver Nanoparticles Significantly Enhanced Thermal Conductivities in Hot-Pressed Electrospun Polyimide Nanocomposites[J]. ACS Applied Materials & Interfaces, 2019, 11(28): 25465-25473. [147] WANG S, AN C, WANG J, et al. Reduce the Sensitivity of CL-20 by Improving Thermal Conductivity Through Carbon Nanomaterials[J]. Nanoscale Research Letters, 2018, 13(1): 85. doi: 10.1186/s11671-018-2496-3 [148] QUAN B, SHI W, ONG S J H, et al. Defect Engineering in Two Common Types of Dielectric Materials for Electromagnetic Absorption Applications[J]. Advanced Functional Materials, 2019, 29(28): 1901236. doi: 10.1002/adfm.201901236 [149] LIU P, GAO S, ZHANG G, et al. Hollow Engineering to Co@N-Doped Carbon Nanocages via Synergistic Protecting-Etching Strategy for Ultrahigh Microwave Absorption[J]. Advanced Functional Materials, 2021, 31(27): 2102812. doi: 10.1002/adfm.202102812 [150] ZHANG F, FENG Y, QIN M, et al. Composite Networks: Stress Controllability in Thermal and Electrical Conductivity of 3D Elastic Graphene-Crosslinked Carbon Nanotube Sponge/Polyimide Nanocomposite[J]. Advanced Functional Materials, 2019, 29(25): 1970173. doi: 10.1002/adfm.201970173 [151] WU Z, CHENG H W, JIN C, et al. Dimensional Design and Core–Shell Engineering of Nanomaterials for Electromagnetic Wave Absorption[J]. Advanced Materials, 2022, 34(11): 2107538. doi: 10.1002/adma.202107538 [152] YANG X, FU K, WU L, et al. Synergistic enhancement of thermal conduction and microwave absorption of silica films based on graphene/chiral PPy/Al2O3 ternary aerogels[J]. Carbon, 2022, 199: 1-12. [153] MOU P, WAN G, WU L, et al, Optimizing impedance matching and interfacial characteristics of aromatic polyimide/graphene by molecular layer deposition for heat-conducting microwave absorption[J]. Journal of Materials Chemistry A, 2023, 11 (8): 4345-4354. [154] YANG X, FAN S, LI Y, et al. Synchronously improved electromagnetic interference shielding and thermal conductivity for epoxy nanocomposites by constructing 3D copper nanowires/thermally annealed graphene aerogel framework[J]. Composites Part A:Applied Science and Manufacturing, 2020, 128: 105670. doi: 10.1016/j.compositesa.2019.105670 [155] LV P, ZHOU X, CHEN S. Hot-Pressed Super-Elastic Graphene Aerogel with Bidirectional Thermal Conduction Properties as Thermal Interface Materials[J]. Materials, 2023, 16(23): 7419. doi: 10.3390/ma16237419 [156] CHEN Y, PANG K, LIU X, et al. Environment-adaptive, anti-fatigue thermal interface graphene foam[J]. Carbon, 2023, 212: 118142. doi: 10.1016/j.carbon.2023.118142 [157] SUN X, WANG Z-Y, WANG Y, et al. Graphene/Polyolefin Elastomer Films as Thermal Interface Materials with High Thermal Conductivity, Flexibility, and Good Adhesion[J]. Chemistry of Materials, 2023, 35(6): 2486-2494 doi: 10.1021/acs.chemmater.2c03730 [158] BALAN A E, AL-SHARER A, LAVASANI E J, et al. Paraffin-Multilayer Graphene Composite for Thermal Management in Electronics[J]. Materials, 2023, 16(6): 2310. doi: 10.3390/ma16062310 [159] SHANG Y, ZHANG D, AN M, et al. Enhanced Thermal Performance of Composite Phase Change Materials Based on Hybrid Graphene Aerogels for Thermal Energy Storage[J]. Materials, 2022, 15(15): 5380. doi: 10.3390/ma15155380 [160] YANG J, YU W, LIU C, et al. Phase change mediated graphene hydrogel-based thermal interface material with low thermal contact resistance for thermal management[J]. Composites Science and Technology, 2022, 219: 109223. doi: 10.1016/j.compscitech.2021.109223 [161] SU M, HAN G, GAO J, et al. Carbon welding on graphene skeleton for phase change composites with high thermal conductivity for solar-to-heat conversion[J]. Chemical Engineering Journal, 2022, 427: 131665. doi: 10.1016/j.cej.2021.131665 [162] JU J J, ZHAO Z B, DU X Y, et al. Thermally conductive and anti-corrosive epoxy composite coatings by synchronously incorporating boron nitride/graphene fillers and polyvinyl pyrrolidone. [J]Ceramics International, 2023, 49 (17, Part B): 28854-28863. -
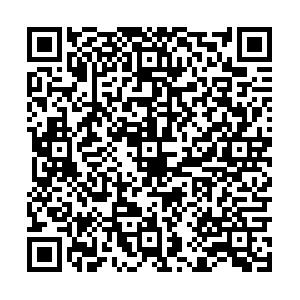
计量
- 文章访问数: 119
- HTML全文浏览量: 52
- 被引次数: 0