Phase-field fracture model of anisotropic materials based on strain energy volumetric-deviatoric split
-
摘要: 断裂相场法被广泛应用于各向同性和复合材料的断裂分析之中。目前,针对各向异性材料和复合材料的断裂问题仍然是断裂相场法的研究重点。本文基于应力拉压的球偏分解方式对弹性应变能进行分解,排除了压缩体积应变能造成的裂纹扩展,并考虑材料拉、压本构关系的非对称情况,建立了一个针对正交各向异性材料断裂问题的相场分析模型。为了验证模型的可靠性,分别对各向同性材料、正交各向异性材料的单边开口板的拉伸和剪切问题进行了分析。对单向纤维夹杂的复合材料板,应用Hashin准则对损伤裂纹驱动力进行了修正,并对不同碳纤维铺设方向复合材料板的拉伸问题进行了模拟。本文建立的模型能够模拟各向异性材料和单向纤维夹杂复合材料的裂纹扩展问题。对于各向同性材料和正交各向异性材料,模拟得到的裂纹扩展路径与现有模型一致,复合材料中裂纹扩展方向和纤维铺设方向平行,预测结果和实验结果吻合良好。Abstract: The phase field method, recognized for its effectiveness in fracture analysis, particularly of isotropic and composite materials, remains a key research focus when considering the intricacies of fractures in anisotropic materials and their composites. In this study, a refined approach to decomposing elastic strain energy was presented. This method excludes the compressive volumetric strain energy influence on crack propagation and accounts for asymmetries in material constitutive relationships under tension and compression. Leveraging this, a tailored phase field analysis model for orthotropic material fractures was constructed. To validate our model's robustness and applicability, we conducted analyses on unidirectional opening plates made of isotropic and orthotropic materials, focusing on tensile and shear boundary conditions. For composite plates with unidirectional fiber reinforcement, the Hashin criterion was incorporated to refine the quantification of damage-induced crack driving forces. This facilitated accurate simulations of tensile behavior in composite plates with varying carbon fiber orientations. Our findings demonstrate the profound capability of our theoretical framework in simulating crack propagation in anisotropic materials and unidirectional fiber-reinforced composites. The predicted crack propagation paths align with those from established models for both isotropic and orthotropic materials, attesting to the model's fidelity. Within composites, we observed a strong alignment between crack propagation and fiber orientation, highlighting a robust correlation between our predictions and experiment results.
-
Key words:
- phase-field method /
- fracture /
- anisotropy /
- composite materials /
- volumetric-deviatoric split
-
表 1 各向同性材料参数
Table 1. Material properties of an isotropic material
E/GPa ν Gc/(GPa∙mm) k 210 0.3 2700 1.0×10−6 Notes: E is the elastic modulus; ν is the Poisson's ratio; Gc is the critical fracture energy density; k is a model parameter that prevents the positive part of the elastic energy density. Property Value C11/GPa 115.8 C12/GPa 39.8 C13/GPa 40.6 C33/GPa 51.4 C44/GPa 20.4 Gc/(GPa∙mm) 1.0×10−3 Notes: C11-C44 is the elastic constants in stiffness matrix; Gc is the critical fracture energy density. 表 3 正交各向异性复合材料板材料参数
Table 3. Material properties of the composite lamina
Property Value E11/GPa 114.8 E22, E33/GPa 11.7 G12/GPa 9.66 ν12 0.21 Gf/( kJ·m−2) 106.3 Gm/( kJ·m−2) 0.2774 Notes: E11 is the longitudinal stiffness of composites; E22 and E33 is the transverse stiffness along the 2- and 3-axis; G12 is the shear stiffness; ν12 is the major poisson’s ratio; Gf is the longitudinal critical energy release rate; Gm is the transverse normal critical energy release rate. Property Value E11/GPa 26.5 E22, E33/GPa 2.6 G12/GPa 1.3 ν12 0.35 Gm/( kJ·m−2) 0.622 Notes: E11 is the longitudinal stiffness of composites; E22 and E33 is the transverse stiffness along the 2- and 3-axis; G12 is the shear stiffness; ν12 is the major poisson’s ratio; Gm is the transverse normal critical energy release rate. -
[1] DOLBOW J E. An extended finite element method with discontinuous enrichment[D]. Evanston: Northwestern University, 1999. [2] KRYSL, Petr, and T. BELYTSCHKO. The Element Free Galerkin method for dynamic propagation of arbitrary 3-D cracks[J]. International Journal for Numerical Methods in Engineering, 1999, 44(6): 767-800. doi: 10.1002/(SICI)1097-0207(19990228)44:6<767::AID-NME524>3.0.CO;2-G [3] SILLING S A. Reformulation of elasticity theory for discontinuities and long-range forces[J]. Journal of the Mechanics and Physics of Solids, 2000, 48: 175-209. doi: 10.1016/S0022-5096(99)00029-0 [4] ZHUANG, X, ZHOU, S, HUYNH, G D, etal. Phase field modeling and computer implementation: a review[J]. Engineering Fracture Mechanics, 2022, 262. [5] HAKIM, V, KARMA, A. Crack path prediction in anisotropic brittle materials[J]. Physical Review Letters, 2004, 95(23): 235501. [6] ABDOLLAHI, A, ARIAS, I. Phase-field modeling of fracture in ferroelectric materials[J]. Archives of Computational Methods in Engineering, 2015, 22(2): 153-181. doi: 10.1007/s11831-014-9118-8 [7] LI, B, PECO, C, MILLÁN, et al. Phase-field modeling and simulation of fracture in brittle materials with strongly anisotropic surface energy[J]. International Journal for Numerical Methods in Engineering, 2014, 102(3-4): 711-727. [8] CLAYTON J D, KNAP J. A geometrically nonlinear phase field theory of brittle fracture[J]. International Journal of Fracture, 2014, 189(2): 139-148. doi: 10.1007/s10704-014-9965-1 [9] CLAYTON J D, KNAP J. Phase field modeling of directional fracture in anisotropic p-olycrystals[J]. Computational Materials Science, 2015, 98: 158-169. doi: 10.1016/j.commatsci.2014.11.009 [10] 张鹏. 纤维增强复合材料破坏过程模拟的相场模型研究[D]. 大连理工大学, 2020.ZHANG Peng. The study of phase field models of failure in fiber reinforced composites[D]. Dalian: Dalian university of technology, 2020. [11] ZHANG P, HU X, BUI T Q, et al. Phase field modeling of fracture in fiber reinforced composite laminate[J]. International Journal of Mechanical Sciences, 2019, 161: 105008. [12] ZHANG P, FENG Y, BUI T Q, et al. Modelling distinct failure mechanisms in composite materials by a combined phase field method[J]. Composite Structures, 2020, 232: 111551. doi: 10.1016/j.compstruct.2019.111551 [13] BLEYER J, ALESSI R. Phase-field modeling of anisotropic brittle fracture including several damage mechanisms[J]. Computer Methods in Applied Mechanics and Engineering, 2018, 336: 213-236. doi: 10.1016/j.cma.2018.03.012 [14] NGUYEN T T, RÉTHORÉ J, BAIETTO M C. Phase field modelling of anisotropic crack propagation[J]. European Journal of Mechanics-A/Solids, 2017, 65: 279-288. doi: 10.1016/j.euromechsol.2017.05.002 [15] TEICHTMEISTER S, KIENLE D, ALDAK-HEEL F, et al. Phase field modeling of fracture in anisotropic brittle solids[J]. International Journal of Non-Linear Mechanics, 2017, 97: 1-21. doi: 10.1016/j.ijnonlinmec.2017.06.018 [16] WU J Y. A unified phase-field theory for the mechanics of damage and quasi-brittle failure[J]. Journal of the Mechanics and Physics of Solids, 2017, 103: 72-99. doi: 10.1016/j.jmps.2017.03.015 [17] WU J Y. A geometrically regularized gradient-damage model with energetic equivalence[J]. Computer Methods in Applied Mechanics and Engineering, 2018, 328: 612-637. doi: 10.1016/j.cma.2017.09.027 [18] WU J Y, NGUYEN V P, ZHOU H, et al. A variationally consistent phase-field anisotropic damage model for fracture[J]. Computer Methods in Applied Mechanics and Engineering, 2020, 358: 112629. doi: 10.1016/j.cma.2019.112629 [19] ZHANG S, JIANG W, TONKS M R. A new phase field fracture model for brittle materials that accounts for elastic anisotropy[J]. Computer Methods in Applied Mechanics and Engineering, 2020, 358: 112643. doi: 10.1016/j.cma.2019.112643 [20] LUO Z, CHEN L, WANG N, et al. A phase-field fracture model for brittle anisotropic materials[J]. Computational Mechanics, 2022, 70(5): 931-943. doi: 10.1007/s00466-022-02192-9 [21] WU J Y, NGUYEN V P, NGUYEN C T, et al. Phase-field modeling of fracture[J]. Advances in applied mechanics, 2020, 53: 1-183. [22] AMOR H, MARIGO J J, MAURINI C. Regularized formulation of the variational brittle fracture with unilateral contact: Numerical experiments[J]. Journal of the Mechanics and Physics of Solids, 2009, 57(8): 1209-1229. doi: 10.1016/j.jmps.2009.04.011 [23] KRISTENSEN P K, MARTÍNEZ-PAÑEDA E. Phase field fracture modelling using quasi-Newton methods and a new adaptive step scheme[J]. Theoretical and Applied Fracture Mechanics, 2020, 107: 102446. doi: 10.1016/j.tafmec.2019.102446 [24] ESPADAS-ESCALANTE J J, VAN DIJK N P, ISAKSSON P. A phase-field model for strength and fracture analyses of fiber-reinforced composites[J]. Composites Science and Technology, 2019, 174: 58-67. doi: 10.1016/j.compscitech.2018.10.031 [25] BORDEN M J, VERHOOSEL C V, SCOTT M A, et al. A phase-field description of dynamic brittle fracture[J]. Computer Methods in Applied Mechanics and Engineering, 2012, 217: 77-95. [26] MIEHE C, HOFACKER M, WELSCHINGER F. A phase field model for rate-independent crack propagation: Robust algorithmic implementation based on operator splits[J]. Computer Methods in Applied Mechanics and Engineering, 2010, 199(45-48): 2765-2778. doi: 10.1016/j.cma.2010.04.011 [27] ZHOU S, RABCZUK T, ZHUANG X. Phase field modeling of quasi-static and dynamic crack propagation: COMSOL implementation and case studies[J]. Advances in Engineering Software, 2018, 122: 31-49. doi: 10.1016/j.advengsoft.2018.03.012 [28] MIEHE C, WELSCHINGER F, HOFACKER M. Thermodynamically consistent phase-field models of fracture: Variational principles and multi-field FE implementations[J]. International journal for numerical methods in engineering, 2010, 83(10): 1273-1311. doi: 10.1002/nme.2861 [29] CAHILL L M A, NATARAJAN S, BORDAS S P A, et al. An experimental/numerical investigation into the main driving force for crack propagation in unidirectional fibre-reinforced composite laminae[J]. Composite Structures, 2014, 107: 119-130. doi: 10.1016/j.compstruct.2013.05.039 [30] HASHIN Z. Fatigue failure criteria for unidirectional fiber composites[J]. Journal of applied mechanics, 1980, 47(4): 329-334. -
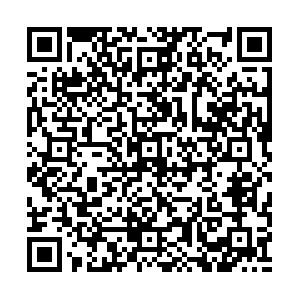
计量
- 文章访问数: 92
- HTML全文浏览量: 83
- 被引次数: 0