Progress in defect modulation of g-C3N4 based materials and its photocatalytic property
-
摘要: 半导体光催化材料已成为有效应对环境污染和能源危机关键技术的核心要素。其中,石墨相氮化碳(g-C3N4)作为一种新兴的高效催化材料展现出了巨大的应用潜力。然而,未改性的g-C3N4存在诸如可见光响应范围有限、活性位点偏少以及光生载流子复合速率高等缺点,严重制约了其实际应用。为了解决上述问题,研究人员采取了多种策略,如设计和开发异质结构、实施缺陷工程和进行形貌调控等。其中,缺陷调控因能有效地调制光催化材料的电子能带结构、延缓载流子的复合和增加表面活性位点等原因备受关注。本文阐述了缺陷修饰的类型、缺陷调控策略,最后对g-C3N4基材料的开发和光催化应用进行了总结并给出了展望。Abstract: Semiconductor photocatalytic materials have become a key factor of photocatalytic technologies to solve environmental pollution and energy crisis. Among them, graphitic phase carbon nitride (g-C3N4) has shown great potential for application as an emerging highly efficient catalytic material. However, the unmodified g-C3N4 has disadvantages such as limited visible light response range, less reactive sites and high photogenerated carrier complexation rate, which severely limit its practical applications. Thus, researchers have adopted various strategies, such as designing and developing heterogeneous structures, defect engineering and morphological modulation to solve the problems mentioned above. Among them, defect modulation has attracted much attention because it can effectively modulate the electronic band structure of photocatalytic materials, delay carrier recombination and increase the surface reactive sites. This paper describes the types of defect modulations, defect modulation strategies, and finally summarizes the development and application of g-C3N4 based photocatalytic materials and gives an outlook.
-
图 6 (a)比较g-C3N4纳米片、g-C3N4纳米片和Fe掺杂g-C3N4纳米片在阳光照射下对水溶液中RhB降解的光催化活性; (b)在阳光照射下,Fe掺杂g-C3N4纳米片在水溶液中降解RhB的高光催化活性示意图[33]
Figure 6. (a)Comparison of the photocatalytic activities of bulk g-C3N4, and pure and Fe-doped g-C3N4 nanosheets for the degradation of RhB in aqueous solution under sunlight irradiation; (b)Schematic diagram of the high photocatalytic activity of Fe-doped g-C3N4 nanosheets for the degradation of RhB in aqueous solution under sunlight irradiation[33]
表 1 氮化碳改性的的各种参数和应用
Table 1. Various parameters and applications of carbon nitride modification
Control strategy Type of defect Add
SubstancesEg/eV BET/(m2 g−1) Application Refer Pre-polymerization adjustment Cv(carbon vacancy) Ar 2.79 160 HER [62] Cv(carbon vacancy) CO2 2.84 147 No oxidization [63] NV(nitrogen vacancy) HNO3 2.78 421.59 HER, Pollutant removal [64] NV, —C≡N(nitrogen and
Cyanide vacanciesNabh4 2.71 53.7 HER [65] NV(nitrogen vacancy) Nabh4 2.66 56.05 No removal [66] Polymerization time adjustment NV(nitrogen vacancy) H2 2.0 - HER [67] NV(nitrogen vacancy) N2 2.78 67.5 No removal [68] NV(nitrogen vacancy) N2 2.07 65.6 Overall water splitting [69] Cv(carbon vacancy) Acetone 2.33 153.78 HER [70] Cv(carbon vacancy) - 2.92 75.24 Nitrogen fixation [71] Notes: Eg—Band gap; BET—Specific surface area; HER—Hydrogen evolution reaction -
[1] ONG W J, TAN L L, NG Y H, et al. Graphitic Carbon Nitride (g-C3N4)-Based Photocatalysts for Artificial Photosynthesis and Environmental Remediation: Are We a Step Closer To Achieving Sustainability?[J]. Chemical reviews, 2016, 116(12): 7159-7329. doi: 10.1021/acs.chemrev.6b00075 [2] MA Y, WANG X, JIA Y, et al. Titanium dioxide-based nanomaterials for photocatalytic fuel generations[J]. Chemical reviews, 2014, 114(19): 9987-10043. doi: 10.1021/cr500008u [3] CHEN X, SHEN S, GUO L, et al. Semiconductor-based photocatalytic hydrogen generation[J]. Chemical reviews, 2010, 110(11): 6503-6570. doi: 10.1021/cr1001645 [4] YAN S C, LI Z S and ZOU Z G. Photodegradation performance of g-C3N4 fabricated by directly heating melamine[J]. Langmuir : the ACS journal of surfaces and colloids, 2009, 25(17) : 10397-10401. [5] ZHANG X, XIE X, WANG H, et al. Enhanced photoresponsive ultrathin graphitic-phase C3N4 nanosheets for bioimaging[J]. Journal of the American Chemical Society, 2013, 135(1): 18-21. doi: 10.1021/ja308249k [6] DONG F, ZHAO Z, XIONG T, et al. In Situ Construction of g-C3N4/ g-C3N4 Metal-Free Heterojunction for Enhanced Visible-Light Photocatalysis[J]. ACS Applied Materials & Interfaces, 2013, 5(21): 11392-11401. [7] 徐杨, 刘成宝, 郑磊之, 等. 高结晶度g-C3N4在光催化领域的研究进展[J]. 材料导报, 2024, 3.1: 1-20.XU Yang, LIU Chengbao, ZHENG Leizhi, et al. Research Progress of High Crystallinity g-C3N4 in photocatalysis[J]. Materials Reports, 2024, 3.1: 1-20. [8] WANG Y, CHEN J, LIU L, et al. Novel metal doped carbon quantum dots/CdS composites for efficient photocatalytic hydrogen evolution[J]. Nanoscale, 2019, 11(4): 1618-1625. doi: 10.1039/C8NR05807E [9] TAY Q, WANG X, ZHAO X, et al. Enhanced visible light hydrogen production via a multiple heterojunction structure with defect-engineered g-C3N4 and two-phase anatase/brookite TiO2[J]. Journal of Catalysis, 2016, 342: 55-62. doi: 10.1016/j.jcat.2016.07.007 [10] FU J, MO Z, CHEN H, et al. Three coordinate nitrogen (N3c) vacancies from in-situ hydrogen bond breaking over polymeric carbon nitride for efficient photocatalysis[J]. Journal of Environmental Chemical Engineering, 2023, 11(2): 109495. doi: 10.1016/j.jece.2023.109495 [11] XIONG Y, CHEN Y, YANG N, et al. WC1−x-Coupled 3D Porous Defective g-C3N4 for Efficient Photocatalytic Overall Water Splitting[J]. Solar RRL, 2019, 3(5): 1800341. doi: 10.1002/solr.201800341 [12] WANG K, FU J and ZHENG Y. Insights into photocatalytic CO2 reduction on C3N4: Strategy of simultaneous B, K co-doping and enhancement by N vacancies[J]. Applied Catalysis B:Environmental, 2019, 254: 270-282. doi: 10.1016/j.apcatb.2019.05.002 [13] WANG X, MAEDA K, THOMAS A, et al. A metal-free polymeric photocatalyst for hydrogen production from water under visible light[J]. Nature materials, 2009, 8(1): 76-80. doi: 10.1038/nmat2317 [14] YU H, SHI R, ZHAO Y, et al. Alkali-Assisted Synthesis of Nitrogen Deficient Graphitic Carbon Nitride with Tunable Band Structures for Efficient Visible-Light-Driven Hydrogen Evolution[J]. Advanced Materials, 2017, 29(16): 1605148. doi: 10.1002/adma.201605148 [15] YANG P, ZHUZHANG H, WANG R, et al. Carbon Vacancies in a Melon Polymeric Matrix Promote Photocatalytic Carbon Dioxide Conversion[J]. Angewandte Chemie (International ed. in English), 2019, 58(4): 1134-1137. doi: 10.1002/anie.201810648 [16] LI F, YUE X, ZHANG D, et al. Targeted regulation of exciton dissociation in graphitic carbon nitride by vacancy modification for efficient photocatalytic CO2 reduction[J]. Applied Catalysis B:Environmental, 2021, 292: 120179. doi: 10.1016/j.apcatb.2021.120179 [17] SHAO W, WANG L, WANG H, et al. Efficient Exciton Dissociation in Heterojunction Interfaces Realizing Enhanced Photoresponsive Performance[J]. The Journal of Physical Chemistry Letters, 2019, 10(11): 2904-2910. doi: 10.1021/acs.jpclett.9b01020 [18] LIU Y, XIAO C, LI Z, et al. Vacancy Engineering for Tuning Electron and Phonon Structures of Two-Dimensional Materials[J]. Advanced Energy Materials, 2016, 6(23): 1600436. doi: 10.1002/aenm.201600436 [19] NIU P, QIAO M, LI Y, et al. Distinctive defects engineering in graphitic carbon nitride for greatly extended visible light photocatalytic hydrogen evolution[J]. Nano Energy, 2018, 44: 73-81. doi: 10.1016/j.nanoen.2017.11.059 [20] IGLESIAS D, GIULIANI A, MELCHIONNA M, et al. N-Doped Graphitized Carbon Nanohorns as a Forefront Electrocatalyst in Highly Selective O2 Reduction to H2O2[J]. Chem, 2018, 4(1): 106-123. doi: 10.1016/j.chempr.2017.10.013 [21] LIN F, WANG T, REN Z, et al. Central nitrogen vacancies in polymeric carbon nitride for boosted photocatalytic H2O2 production[J]. Journal of Colloid and Interface Science, 2023, 636: 223-229. doi: 10.1016/j.jcis.2023.01.036 [22] XIE Y, LI Y, HUANG Z, et al. Two types of cooperative nitrogen vacancies in polymeric carbon nitride for efficient solar-driven H2O2 evolution[J]. Applied Catalysis B:Environmental, 2020, 265: 118581. doi: 10.1016/j.apcatb.2019.118581 [23] DONG G, JACOBS D L, ZANG L, et al. Carbon vacancy regulated photoreduction of NO to N2 over ultrathin g-C3N4 nanosheets[J]. Applied Catalysis B:Environmental, 2017, 218: 515-524. doi: 10.1016/j.apcatb.2017.07.010 [24] LIU M, ZHANG D, HAN J, et al. Adsorption enhanced photocatalytic degradation sulfadiazine antibiotic using porous carbon nitride nanosheets with carbon vacancies[J]. Chemical Engineering Journal, 2020, 382: 123017. doi: 10.1016/j.cej.2019.123017 [25] LIANG Q, LI Z, HUANG Z-H, et al. Hydrogen Evolution: Holey Graphitic Carbon Nitride Nanosheets with Carbon Vacancies for Highly Improved Photocatalytic Hydrogen Production[J]. Advanced Functional Materials, 2015, 25(44): 6952-6952. doi: 10.1002/adfm.201570285 [26] WANG J, HUANG J, XIE H, et al. Synthesis of g-C3N4/TiO2 with enhanced photocatalytic activity for H2 evolution by a simple method[J]. International Journal of Hydrogen Energy, 2014, 39(12): 6354-6363. doi: 10.1016/j.ijhydene.2014.02.020 [27] ZHENG Y, LIN L, WANG B, et al. Graphitic Carbon Nitride Polymers toward Sustainable Photoredox Catalysis[J]. Angewandte Chemie International Edition, 2015, 54(44): 12868-12884. doi: 10.1002/anie.201501788 [28] XU J, BRENNER T, CHEN Z, et al. Upconversion-Agent Induced Improvement of g-C3N4 Photocatalyst under Visible Light[J]. ACS applied materials & interfaces, 2014, 6. [29] ZHANG M, BAI X, LIU D, et al. Enhanced catalytic activity of potassium-doped graphitic carbon nitride induced by lower valence position[J]. Applied Catalysis B:Environmental, 2015, 164: 77-81. doi: 10.1016/j.apcatb.2014.09.020 [30] HU S, LI F, FAN Z, et al. Band gap-tunable potassium doped graphitic carbon nitride with enhanced mineralization ability[J]. Dalton Transactions, 2015, 44(3): 1084-1092. doi: 10.1039/C4DT02658F [31] XIONG T, CEN W, ZHANG Y, et al. Bridging the g-C3N4 Interlayers for Enhanced Photocatalysis[J]. ACS Catalysis, 2016, 6(4): 2462-2472. doi: 10.1021/acscatal.5b02922 [32] WANG N, WANG J, HU J, et al. Design of Palladium-Doped g-C3N4 for Enhanced Photocatalytic Activity toward Hydrogen Evolution Reaction[J]. ACS Applied Energy Materials, 2018, 1(6): 2866-2873. doi: 10.1021/acsaem.8b00526 [33] TONDA S, KUMAR S, KANDULA S, et al. Fe-doped and -mediated graphitic carbon nitride nanosheets for enhanced photocatalytic performance under natural sunlight[J]. Journal of Materials Chemistry A, 2014, 2(19): 6772-6780. doi: 10.1039/c3ta15358d [34] ZHANG Y, MORI T, YE J, et al. Phosphorus-doped carbon nitride solid: enhanced electrical conductivity and photocurrent generation[J]. Journal of the American Chemical Society, 2010, 132(18): 6294-6295. doi: 10.1021/ja101749y [35] ZHANG L, CHEN X, GUAN J, et al. Facile synthesis of phosphorus doped graphitic carbon nitride polymers with enhanced visible-light photocatalytic activity[J]. Materials Research Bulletin, 2013, 48(9): 3485-3491. doi: 10.1016/j.materresbull.2013.05.040 [36] HU S, MA L, YOU J, et al. A simple and efficient method to prepare a phosphorus modified g-C3N4 visible light photocatalyst[J]. RSC Advances, 2014, 4(41): 21657-21663. doi: 10.1039/C4RA02284J [37] LIU G, NIU P, SUN C, et al. Unique Electronic Structure Induced High Photoreactivity of Sulfur-Doped Graphitic C3N4[J]. Journal of the American Chemical Society, 2010, 132(33): 11642-11648. doi: 10.1021/ja103798k [38] MA L, HU S, LI P, et al. In situ synthesis of sulfur doped carbon nitride with enhanced photocatalytic performance using DBD plasma treatment under H2S atmosphere[J]. Journal of Physics and Chemistry of Solids, 2018, 118: 166-171. doi: 10.1016/j.jpcs.2018.03.017 [39] FAN Q, LIU J, YU Y, et al. A simple fabrication for sulfur doped graphitic carbon nitride porous rods with excellent photocatalytic activity degrading RhB dye[J]. Applied Surface Science, 2017, 391: 360-368. doi: 10.1016/j.apsusc.2016.04.055 [40] ROOSE B, PATHAK S and STEINER U. Doping of TiO2 for sensitized solar cells[J]. Chemical Society Reviews, 2015, 44(22): 8326-8349. doi: 10.1039/C5CS00352K [41] NASIR M, BAGWASI S, JIAO Y, et al. Characterization and activity of the Ce and N co-doped TiO2 prepared through hydrothermal method[J]. Chemical Engineering Journal, 2014, 236: 388-397. doi: 10.1016/j.cej.2013.09.095 [42] SHEN X-Z, LIU Z-C, XIE S-M, et al. Degradation of nitrobenzene using titania photocatalyst co-doped with nitrogen and cerium under visible light illumination[J]. Journal of Hazardous Materials, 2009, 162(2): 1193-1198. [43] HU S, MA L, YOU J, et al. Enhanced visible light photocatalytic performance of g-C3N4 photocatalysts co-doped with iron and phosphorus[J]. Applied Surface Science, 2014, 311: 164-171. doi: 10.1016/j.apsusc.2014.05.036 [44] ZHANG S, LI J, ZENG M, et al. Bandgap Engineering and Mechanism Study of Nonmetal and Metal Ion Codoped Carbon Nitride: C+Fe as an Example[J]. Chemistry – A European Journal, 2014, 20(31): 9805-9812. doi: 10.1002/chem.201400060 [45] HU S, MA L, XIE Y, et al. Hydrothermal synthesis of oxygen functionalized S–P codoped g-C3N4 nanorods with outstanding visible light activity under anoxic conditions[J]. Dalton Transactions, 2015, 44(48): 20889-20897. doi: 10.1039/C5DT04035C [46] MA H, ZHAO S, LI S, et al. A facile approach to synthesizing S–Co–O tridoped g-C3N4 with enhanced oxygen-free photocatalytic performance via a hydrothermal post-treatment[J]. RSC Advances, 2015, 5(97): 79585-79592. doi: 10.1039/C5RA14081A [47] DI J, JIEXIANG X, LI X, et al. Constructing confined surface carbon defects in ultrathin graphitic carbon nitride for photocatalytic free radical manipulation[J]. Carbon, 2016, 107. [48] XIA Y, YANG H, HO W, et al. Promoting the photocatalytic NO oxidation activity of hierarchical porous g-C3N4 by introduction of nitrogen vacancies and charge channels[J]. Applied Catalysis B:Environmental, 2024, 344: 123604. doi: 10.1016/j.apcatb.2023.123604 [49] LIAO J, CUI W, LI J, et al. Nitrogen defect structure and NO+ intermediate promoted photocatalytic NO removal on H2 treated g-C3N4[J]. Chemical Engineering Journal, 2020, 379: 122282. doi: 10.1016/j.cej.2019.122282 [50] ZHAO D, DONG C-L, WANG B, et al. Synergy of Dopants and Defects in Graphitic Carbon Nitride with Exceptionally Modulated Band Structures for Efficient Photocatalytic Oxygen Evolution[J]. Advanced Materials, 2019, 31(43): 1903545. doi: 10.1002/adma.201903545 [51] GU Z, CUI Z, WANG Z, et al. Carbon vacancies and hydroxyls in graphitic carbon nitride: Promoted photocatalytic NO removal activity and mechanism[J]. Applied Catalysis B:Environmental, 2020, 279: 119376. doi: 10.1016/j.apcatb.2020.119376 [52] LIU G, ZHAO G, ZHOU W, et al. In Situ Bond Modulation of Graphitic Carbon Nitride to Construct p–n Homojunctions for Enhanced Photocatalytic Hydrogen Production[J]. Advanced Functional Materials, 2016, 26(37): 6822-6829. doi: 10.1002/adfm.201602779 [53] TIAN J, WANG D, LI S, et al. KOH-Assisted Band Engineering of Polymeric Carbon Nitride for Visible Light Photocatalytic Oxygen Reduction to Hydrogen Peroxide[J]. ACS Sustainable Chemistry & Engineering, 2020, 8(1): 594-603. [54] LIN W, LU K, ZHOU S, et al. Defects remodeling of g-C3N4 nanosheets by fluorine-containing solvothermal treatment to enhance their photocatalytic activities[J]. Applied Surface Science, 2019, 474: 194-202. doi: 10.1016/j.apsusc.2018.03.140 [55] NIU P, LIU G and CHENG H-M. Nitrogen Vacancy-Promoted Photocatalytic Activity of Graphitic Carbon Nitride[J]. The Journal of Physical Chemistry C, 2012, 116(20): 11013-11018. doi: 10.1021/jp301026y [56] KANG Y, YANG Y, YIN L-C, et al. An Amorphous Carbon Nitride Photocatalyst with Greatly Extended Visible-Light-Responsive Range for Photocatalytic Hydrogen Generation[J]. Advanced Materials, 2015, 27(31): 4572-4577. doi: 10.1002/adma.201501939 [57] HAN Q, CHENG Z, WANG B, et al. Significant Enhancement of Visible-Light-Driven Hydrogen Evolution by Structure Regulation of Carbon Nitrides[J]. ACS nano, 2018, 12(6): 5221-5227. doi: 10.1021/acsnano.7b08100 [58] DENG Y, ZHOU Z, ZENG H, et al. Phosphorus and kalium co-doped g-C3N4 with multiple-locus synergies to degrade atrazine: Insights into the depth analysis of the generation and role of singlet oxygen[J]. Applied Catalysis B:Environmental, 2023, 320: 121942. doi: 10.1016/j.apcatb.2022.121942 [59] CHEN Z, CHEN W, LIAO G, et al. Flexible construct of N vacancies and hydrophobic sites on g-C3N4 by F doping and their contribution to PFOA degradation in photocatalytic ozonation[J]. Journal of Hazardous Materials, 2022, 428: 128222. doi: 10.1016/j.jhazmat.2022.128222 [60] CUI Y, ZHANG G, LIN Z, et al. Condensed and low-defected graphitic carbon nitride with enhanced photocatalytic hydrogen evolution under visible light irradiation[J]. Applied Catalysis B:Environmental, 2016, 181: 413-419. doi: 10.1016/j.apcatb.2015.08.018 [61] TU W, XU Y, WANG J, et al. Investigating the Role of Tunable Nitrogen Vacancies in Graphitic Carbon Nitride Nanosheets for Efficient Visible-Light-Driven H2 Evolution and CO2 Reduction[J]. ACS Sustainable Chemistry & Engineering, 2017, 5(8): 7260-7268. [62] GHOLIPOUR M R, BéLAND F, DO T O J A S C, et al. Post-Calcined Carbon Nitride Nanosheets as an Efficient Photocatalyst for Hydrogen Production under Visible Light Irradiation[J]. 2017, 5: 213-220. [63] LI Y, HO W, LV K, et al. Carbon vacancy-induced enhancement of the visible light-driven photocatalytic oxidation of NO over g-C3N4 nanosheets[J]. Applied Surface Science, 2018, 430: 380-389. doi: 10.1016/j.apsusc.2017.06.054 [64] CHE H, LIU L, CHE G, et al. Control of energy band, layer structure and vacancy defect of graphitic carbon nitride by intercalated hydrogen bond effect of NO3− toward improving photocatalytic performance[J]. Chemical Engineering Journal, 2019, 357: 209-219. doi: 10.1016/j.cej.2018.09.112 [65] WEN Y, QU D, AN L, et al. Defective g-C3N4 Prepared by the NaBH4 Reduction for High-Performance H2 Production[J]. ACS Sustainable Chemistry & Engineering, 2019, 7(2): 2343-2349. [66] CAO J, ZHANG J, DONG X A, et al. Defective borate-decorated polymer carbon nitride: Enhanced photocatalytic NO removal, synergy effect and reaction pathway[J]. Applied Catalysis B:Environmental, 2019, 249: 266-274. doi: 10.1016/j.apcatb.2019.03.012 [67] TAY Q, KANHERE P, NG C F, et al. Defect Engineered g-C3N4 for Efficient Visible Light Photocatalytic Hydrogen Production[J]. Chemistry of Materials, 2015, 27(14): 4930-4933. doi: 10.1021/acs.chemmater.5b02344 [68] WANG Z, HUANG Y, CHEN M, et al. Roles of N-Vacancies over Porous g-C3N4 Microtubes during Photocatalytic NOx Removal[J]. ACS Applied Materials & Interfaces, 2019, 11(11): 10651-10662. [69] ZENG Y, LI H, LUO J, et al. Sea-urchin-structure g-C3N4 with narrow bandgap (˜2.0 eV) for efficient overall water splitting under visible light irradiation[J]. Applied Catalysis B:Environmental, 2019, 249: 275-281. doi: 10.1016/j.apcatb.2019.03.010 [70] JIANG L, LI J, WANG K, et al. Low boiling point solvent mediated strategy to synthesize functionalized monolayer carbon nitride for superior photocatalytic hydrogen evolution[J]. Applied Catalysis B:Environmental, 2020, 260: 118181. doi: 10.1016/j.apcatb.2019.118181 [71] CAO S, FAN B, FENG Y, et al. Sulfur-doped g-C3N4 nanosheets with carbon vacancies: General synthesis and improved activity for simulated solar-light photocatalytic nitrogen fixation[J]. Chemical Engineering Journal, 2018, 353: 147-156. doi: 10.1016/j.cej.2018.07.116 [72] MENG F, TIAN W, TIAN Z, et al. Enhanced photocatalytic organic pollutant degradation and H2 evolution reaction over carbon nitride nanosheets: N defects abundant materials[J]. Science of The Total Environment, 2022, 851: 158360. doi: 10.1016/j.scitotenv.2022.158360 [73] BAI X, LI M, LI J, et al. Chemical Cutting of Network Nodes in Polymeric Carbon Nitride for Enhanced Visible-Light Photocatalytic Hydrogen Generation[J]. ACS Applied Nano Materials, 2022, 5(1): 691-701. doi: 10.1021/acsanm.1c03419 [74] DUAN Y, WANG Y, GAN L, et al. Amorphous Carbon Nitride with Three Coordinate Nitrogen (N3C) Vacancies for Exceptional NOx Abatement in Visible Light[J]. Advanced Energy Materials, 2021, 11(19): 2004001. doi: 10.1002/aenm.202004001 [75] ZHANG D, GUO Y and ZHAO Z. Porous defect-modified graphitic carbon nitride via a facile one-step approach with significantly enhanced photocatalytic hydrogen evolution under visible light irradiation[J]. Applied Catalysis B:Environmental, 2018, 226: 1-9. doi: 10.1016/j.apcatb.2017.12.044 [76] GUO S, DENG Z, LI M, et al. Phosphorus-Doped Carbon Nitride Tubes with a Layered Micro-nanostructure for Enhanced Visible-Light Photocatalytic Hydrogen Evolution[J]. Angewandte Chemie International Edition, 2016, 55(5): 1830-1834. doi: 10.1002/anie.201508505 [77] 曹一达, 刘成宝, 陈丰, 等. CeO2/BiOI/ g-C3N4三相复合材料的制备及可见光催化降解RhB性能研究 材料导报[J]. 2023, 37(03): 136-142.CAO Yida, LIU Chengbao, CHEN Feng , et al. Preparation of CeO2/BiOI/g-C3N4 Composite and Its Photocatalytic Degradation Property for RhB Under Visible Light[J]. Materials Reports, 2023, 37(03): 136-142. [78] 于巧玲, 刘成宝, 金涛. 等. CuS/CQDs/ g-C3N4复合材料的合成及其光催化性能研究 材料导报[J]. 2024.3. 1: 1-15.YU Qiaoling, LIU Chengbao, JIN Tao, et al. Synthesis and photocatalytic properties of CuS/CQDs/g-C3N4[J]. Materials Reports, 2024.3. 1: 1-15. [79] FANG H-X, GUO H, NIU C-G, et al. Hollow tubular graphitic carbon nitride catalyst with adjustable nitrogen vacancy: Enhanced optical absorption and carrier separation for improving photocatalytic activity[J]. Chemical Engineering Journal, 2020, 402: 126185. doi: 10.1016/j.cej.2020.126185 [80] ZHU J, CARABINEIRO S A C, SHAN D, et al. Oxygen activation sites in gold and iron catalysts supported on carbon nitride and activated carbon[J]. Journal of Catalysis, 2010, 274(2): 207-214. doi: 10.1016/j.jcat.2010.06.018 [81] HONG Z, SHEN B, CHEN Y, et al. Enhancement of photocatalytic H2 evolution over nitrogen-deficient graphitic carbon nitride[J]. Journal of Materials Chemistry A, 2013, 1(38): 11754-11761. doi: 10.1039/c3ta12332d [82] XU J, WANG Z and ZHU Y. Enhanced Visible-Light-Driven Photocatalytic Disinfection Performance and Organic Pollutant Degradation Activity of Porous g-C3N4 Nanosheets[J]. ACS Applied Materials & Interfaces, 2017, 9(33): 27727-27735. [83] LIU H, MA S, SHAO L, et al. Defective engineering in graphitic carbon nitride nanosheet for efficient photocatalytic pathogenic bacteria disinfection[J]. Applied Catalysis B:Environmental, 2020, 261: 118201. doi: 10.1016/j.apcatb.2019.118201 [84] ZHAO H, YU H, QUAN X, et al. Fabrication of atomic single layer graphitic-C3N4 and its high performance of photocatalytic disinfection under visible light irradiation[J]. Applied Catalysis B:Environmental, 2014, 152-153: 46-50. doi: 10.1016/j.apcatb.2014.01.023 [85] XU J, WANG Z and ZHU Y. Highly efficient visible photocatalytic disinfection and degradation performances of microtubular nanoporous g-C3N4 via hierarchical construction and defects engineering[J]. Journal of Materials Science & Technology, 2020, 49: 133-143. -
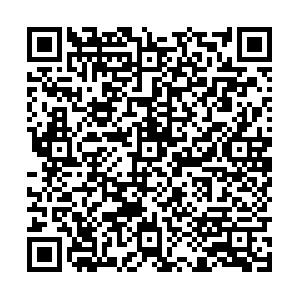
计量
- 文章访问数: 53
- HTML全文浏览量: 18
- 被引次数: 0