Research progress of cathode materials for aluminum-ion batteries
-
摘要: 铝离子电池具有高理论比容量、安全性高、低成本、原料来源充足等优势,被认为是锂离子电池的潜在替代品。但正极材料固有特性的不足极大地限制了铝离子电池的进一步发展。本文总结了正极材料在铝离子电池应用领域所发挥的重要作用,综述了铝离子电池正极材料的作用机制和研究进展,概述了碳基、过渡金属氧化物、硫化物、有机材料、金属有机骨架化合物等正极材料对铝离子电池电化学性能的影响。最后,对正极材料在铝离子电池领域亟需解决的问题进行了探讨,对未来铝离子电池正极材料的发展方向提出了展望。Abstract: With high theoretical specific capacity, high safety, low cost, and sufficient raw material sources, aluminum-ion batteries have been regarded as potential alternatives to lithium-ion batteries. However, the shortcomings of the inherent characteristics of the cathode material have greatly limited the further development of aluminum-ion batteries. In this paper, the important role of cathode materials in the application filed of aluminum ion battery was summarized, the mechanism of action and research progress of aluminum ion electrode materials were reviewed, and the effects of various cathode materials such as carbon-based, transition metal oxides and sulfides, organic materials and metal-organic skeletal compounds on the electrochemical performance of aluminum ion batteries were summarize. Finally, the problems that need to be solved urgently in the field of positive electrode materials for aluminum ion batteries are discussed, and the future development direction of positive electrode materials for aluminum ion batteries is proposed.
-
Key words:
- Al ion batteries /
- cathode material /
- reaction mechanism /
- electrochemical performance /
- new energy
-
图 4 SGN的电化学性能。(a) NG和SGN在不同电流密度下的循环充放电曲线;(b) 不同电流密度下的速率性能;(c) SGN的循环性能[31]
Figure 4. The electrochemical performance of the SGN. (a) the charge and discharge curves of the cycle of NG and SGN at different current densities;(b) The rate performance test at different current densities; (c) The cycling performance of SGN [31]
图 5 (a) 原始EG3 K独立膜的SEM图像;(b), (c) 独立EG3 K阴极膜在充满电状态时的表面和截面SEM图像;(d), (e) EG3 K正极材料在充满电状态时的TEM图像[14]
Figure 5. (a) SEM image of the original EG3 K free-standing film; (b), (c) surface and cross-section SEM images of the free-standing EG3 K cathode film at the fully charged state; (d), (e) TEM images of the EG3 K cathode material at the fully charged state [14]
图 9 GA薄膜的形貌和结构表征。(a) 低倍率SEM图像;(b) 高倍率SEM图像;(c) GA TEM图像;(d), (e)低倍率和高倍率GA薄膜截面SEM图像;(f) GA薄膜俯视图SEM图像 [40]
Figure 9. Morphological and structural characterizations of GA films.(a) Low- magnification SEM images; (b) High-magnification SEM images; (c) TEM image of GA; (d), (e) Low and high magnification cross-section SEM images of GA films; (f) Top-view SEM images of GA films[40]
图 11 (a) 少层石墨烯纳米片(FLG)正极制备过程示意图;(b) FLG的平坦表面和高结晶度(插入衍射点)的TEM图像;(c) 单层(左)、双层(中)和五层(右)FLG纳米片的HRTEM图像;(d) FLG薄膜的横切面;(e) FLG膜的俯视SEM图像[27]
Figure 11. (a) Scheme describing Low-layer graphene nanosheets (FLG) cathode formation; (b) TEM image showing the flat surface, and high crystallinity (inset diffraction spots) of FLG; (c) HRTEM images of single-layer (left), double-layer (middle), and five-layer (right) FLG nanosheets; (d) Cross-section of FLG ; (e) top-view SEM images of FLG film[27]
图 12 FLG AIBs的电化学性能。(a) 倍率性能;(b) 不同循环下的充放电曲线;(c) 10 A/g电流密度下的循环性能[27]
Figure 12. Electrochemical characterization of FLG-AIBs. (a) The rate capability of FLG-AIBs obtained at different current densities; (b) The charge–discharge curves of Al/GA at different cycle; (c) The cycling stability tested at 10 A/g [27]
图 13 (a) 多壁碳纳米管(MWCNTs)不能储存AlCl4−示意图;(b) 柔性多壁碳纳米管(UCNTs)储存AlCl4−示意图;(c) UCNTs制备的可弯曲柔性电极;(d), (e) 优化后UCNTs的SEM图和TEM图;(f) 优化后UCNTs外缘的HR-TEM图像[45]
Figure 13. (a) Multi-walled carbon nanotubes (MWCNTs) which cannot store AlCl4−; (b) The unzipped multiwalled carbon nanotubes (UCNTs) to store chloroaluminate anions; (c) The digital photograph of a bendable flexible electrode prepared using the optimized unzipped carbon nanotubes; (d), (e) SEM image and TEM image of the optimized unzipped carbon nanotubes; (f) HR-TEM image for the external edge of the optimized unzipped carbon nanotubes[45]
图 15 (a) 碳纳米卷的合成步骤示意图;(b) 高温裂解温度达到1000℃时,中间产物的SEM图像;(c), (d)碳纳米卷的SEM图像;(e) 样品的TEM图像;(f) 高倍率TEM,插图中IFFT图像显示了单个石墨烯层[46]
Figure 15. (a) Schematic illustration of the fabrication steps for the synthesis of carbon nanoscrolls; (b) SEM image of the intermediate state product when the annealing temperature reaches 1000℃; (c), (d) SEM images of the as-prepared carbon nanoscrolls; (e) Typical TEM image of the sample; (f) High-resolution TEM. The IFFT image in the illustration exhibits a single graphene layer. [46]
图 19 (a) Co3S4微球的XRD图谱;(b) SEM图像;(c), (d) TEM图像。其中(b)中的插图是Co3S4微球的EDS光谱。(d)中的插图是Co3S4微球的HRTEM图像[50]
Figure 19. (a) XRD pattern; (b) SEM image; (c), (d) TEM image of the Co3S4 microspheres. Inset in (b) is an EDS spectrum of the Co3S4 microspheres. Inset in (d) is an HRTEM image of the Co3S4 microspheres[50]
图 20 (a) Co3S4正极的初始充放电曲线;(b) 循环性能;(c) Co3S4正极用于AIBs与其他代表性金属氧化物/硫化物的循环性能的比较;(d) 不同电流密度下的倍率性能[50]
Figure 20. (a) Initial discharge/charge curves of a Co3S4 cathode; (b) Cycling performance of a Co3S4 cathode; (c) Comparison of the cycling performance of Co3S4 cathodes with other representative metal oxides/sulfides for AIBs; (d) Rate performance of a Co3S4 cathode at different current densities[50]
图 21 MoSe2 HNRA正极应用于AIBs的示意图。(a) 通过掠角沉积系统生长Mo HNRA;(b) 等离子体辅助硒化过程;(c)基于MoSe2 HNRA的AIB的工作机制[52]
Figure 21. Schematic illustration of the MoSe2 HNRA toward AIBs.(a) Growth of Mo HNRAs by the glancing angle depositing system;(b) The plasma-assisted selenization process; (c) Working mechanism of the MoSe2 HNRA-based AIBs[52]
图 25 (a) 三种PQ化合物的结构式—PQ单体(PQ-Ref)、线性PQ三聚体(PQ-Lin)和PQ三角形(PQ-Δ); (b) PQ-Δ(蓝色)的电化学氧化还原化学及其示意图; (c) PQ-Δ及PQ衍生物的循环性能; (d)PQ-Δ倍率性能; (e) 2 A/g的电流密度下PQ-Δ的循环性能; (f) 0.2 A/g电流密度下PQ-Δ-HY的循环性能;(g) PQ-Δ-HY的倍率性能[63]
Figure 25. (a) Structural formulae of three PQ compounds—the PQ monomer (PQ-Ref), the linear PQ trimer (PQ-Lin) and the PQ triangle (PQ-Δ); (b) Electrochemical redox chemistry of PQ-Δ (blue) and its schematic representation; (c) Cycling performances of PQ derivatives; (d) Rate capability measurement of PQ-Δ; (e) Extended cycling test of PQ-Δ; (f) Cycling performance of PQ-Δ-HY at the current rate of 0.2 A/g (2C); (g) Rate capability measurement of PQ-Δ-HY[63]
图 26 (a) PTCDA聚合反应机制; (b) PTCDA正极在充放电过程中的电化学氧化还原反应; (c) 倍率能力; (d) 循环稳定性; (e) 软包电池原理图; (f) AIBs中不同有机正极材料的比较[64]
Figure 26. (a) The polymerization reaction mechanism of PTCDA; (b) The electrochemical redox reaction of PTCDA in the process of charge and discharge; (c) The rate capability; (d) Cycling stability; (e) Schematic diagram of soft pack battery; (f) Comparison of different organic positive electrode materials in AlBs[64]
图 27 (a), (b) H2TPP和H2TCPP的分子结构; (c) H2TPP-xAlCl2模型正的DFT计算得出的反应过程的吉布斯自由能; (d)采用分子静电电位法对H2TPP电极进行两步氧化还原; (e) H2TPP和H2TCPP的循环性能[65]
Figure 27. (a), (b) Molecular structure of H2TPP and H2TCPP; (c) The Gibbs free energy of the reaction process from DFT calculations for H2TPP-xAlCl2 model positive; (d) Two-step redox process of H2TPP electrode obtained by molecular electrostatic potential (MESP) method; (e) Cycling stability of H2TPP and H2TCPP[65]
图 28 (a) 四氰基乙烯(TCNE)、四氰基醌二甲烷(TCNQ)和四(4-氰基苯基)甲烷(TCPM)的化学结构; (b) ,(c) 利用密度泛函理论(DFT)计算的TCNE、TCNQ和TCPM的分子静电势(MESP)图、最低未占据分子轨道(LUMO)和最高占据分子轨道(HOMO); (d) TCNQ的充放电机制; (e) 带乙炔黑改性隔膜和未带的Al-TCNQ 电池中的循环性能[60]
Figure 28. (a) Chemical structures of tetracyanoethylene (TCNE), tetracyanoquinodimethane (TCNQ), and tetrakis(4-cyanophenyl)methane (TCPM);(b, c) The molecular electrostatic potential (MESP) map, the lowest unoccupied molecular orbital (LUMO) and the highest occupied molecular orbital (HOMO) of TCNE, TCNQ, and TCPM calculated using density functional theory (DFT); (d) The electrochemical redox reactions based on two-electron reaction; (e) Cycling performance of TCNQ in the Al batteries with and without acetylene black modified separator[60]
图 33 (a) 不同电流密度下Ti3C2@CTAB-Se的充放电曲线;(b) 循环性能;(c) 本文与其他文献中铝电池正极材料在容量和电压中的比较[76]
Figure 33. (a) The charge–discharge curves of Ti3C2@CTAB-Se at different current densities; (b) The cycles performance; (c) A comparison of capacity and voltage of aluminum batteries cathode materials in this work and literature[76]
图 35 (a) 中空纳米管碳包覆碲(Te@CT)的制备示意图;(b) Te@CW SEM图;(c) Te@CT SEM图;(d), (e) Te@CW TEM图;(f), (g) Te@CT TEM图[81]
Figure 35. (a) The preparation schematic diagram of hollow nanotube carbon-coated tellurium (Te@CT); (b) SEM diagram of Te@CW; (c) SEM diagram of Te@CT; (d), (e) TEM diagram of Te@CW; (f), (g) TEM diagram of Te@CT[81]
图 37 N-PC-rGO-Te合成过程和形态表征。(a) N-PC-Te和N-PC-rGO-Te复合电极的合成过程示意图;(b-e) ZIF-67,N-PC-27,N-PC-Te和N-PC-rGO-Te的SEM图像,插图是相应的单个十二面体;(f-i) 相应的透射电镜图像[82]
Figure 37. Synthesis process and morphology characterizations. (a) Schematic illustration of the synthetic procedure of the N-PC-Te and N-PC-rGO-Te composite electrodes; (b-e) SEM images of ZIF-67, N-PC-27, N-PC-Te and N-PC-rGO-Te, and
N-PC—N-doped porous carbon; rGO—reduced graphene oxide inset is the corresponding single dodecahedron; (f-i) The corresponding TEM images[82]
图 38 N-PC-rGO-Te正极的电化学性能。(a), (b)不同电流密度下的速率性能及相应的充放电曲线;(c) 500 mA/g电流密度下循环性能[82]
Figure 38. The electrochemical performances of N-PC-rGO-Te positive electrode. (a), (b) The rate performance and corresponding charge/discharge curves at various current densities; (c) The cycling performance at a current density of 500 mA/g [82]
-
[1] LI C, HOU C-C, CHEN L, et al. Rechargeable Al-ion batteries[J]. EnergyChem, 2021, 3(2): 100049. doi: 10.1016/j.enchem.2020.100049 [2] LI H, LI L, LIN R-B, et al. Porous metal-organic frameworks for gas storage and separation: Status and challenges[J]. EnergyChem, 2019, 1(1): 100006. doi: 10.1016/j.enchem.2019.100006 [3] HUANG Y, LI J. Key challenges for grid-scale lithium-ion battery energy storage[J]. Advanced Energy Materials, 2022, 12(48): 2202197. doi: 10.1002/aenm.202202197 [4] 李仲明, 李斌, 冯东, 等. 锂离子电池正极材料研究进展[J]. 复合材料学报, 2022, 39(2): 513-527.LI Zhongming, LI Bin, FENG Dong, et al. Research progress of cathode materials for lithium-ion battery[J]. Acta Materiae Compositae Sinica, 2022, 39(2): 513-527 (in Chinese). [5] ZHANG J, LIU T, CHENG X, et al. Development status and future prospect of non-aqueous potassium ion batteries for large scale energy storage[J]. Nano Energy, 2019, 60: 340-361. doi: 10.1016/j.nanoen.2019.03.078 [6] PIRAYESH P, JIN E, WANG Y, et al. Na metal anodes for liquid and solid-state Na batteries[J]. Energy & Environmental Science, 2024, DOI: https://doi.org/10.1039/D3EE03477A. [7] WANG H, MATIOS E, LUO J, et al. Combining theories and experiments to understand the sodium nucleation behavior towards safe sodium metal batteries[J]. Chemical Society Reviews, 2020, 49(12): 3783-3805. doi: 10.1039/D0CS00033G [8] ZHENG X, GU Z, FU J, et al. Knocking down the kinetic barriers towards fast-charging and low-temperature sodium metal batteries[J]. Energy & Environmental Science, 2021, 14(9): 4936-4947. [9] 陈亚鑫, 蔡雅菱, 曹振江, 等. 软@硬复合炭结构设计及其协同电化学储钾[J]. 复合材料学报, 2023, 40(1): 192-200.CHEN Yaxin, CAI Yaling, CAO Zhenjiang, et al. Hard@soft composite carbon anodes towards synergistic potassium storage[J]. Acta Materiae Compositae Sinica, 2023, 40(1): 192-200 (in Chinese). [10] LIU Q, DENG W, SUN C-F. A potassium–tellurium battery[J]. Energy Storage Materials, 2020, 28: 10-6. doi: 10.1016/j.ensm.2020.02.021 [11] DING J, ZHANG H, FAN W, et al. Potassium–sulfur batteries: Review of emerging potassium–sulfur batteries (Adv. Mater. 23/2020)[J]. Advanced Materials, 2020, 32(23): 2070174. doi: 10.1002/adma.202070174 [12] HUANG X L, GUO Z, DOU S X, et al. Rechargeable potassium–selenium batteries[J]. Advanced Functional Materials, 2021, 31(29): 2102326. doi: 10.1002/adfm.202102326 [13] PARK J, XU Z-L, YOON G, et al. Calcium-ion batteries: stable and high-power calcium-ion batteries enabled by calcium intercalation into graphite (Adv. Mater. 4/2020)[J]. Advanced Materials, 2020, 32(4): 2070029. doi: 10.1002/adma.202070029 [14] YAO Z, HEGDE V I, ASPURU-GUZIK A, et al. Discovery of calcium-metal alloy anodes for reversible Ca-ion batteries[J]. Advanced Energy Materials, 2019, 9(9): 1802994. doi: 10.1002/aenm.201802994 [15] 涂天成, 李龙燕, 代启航. 水系镁离子电池研究进展[J]. 复合材料学报, 2023, 40(7): 3756-3770.TU Tiancheng, LI Longyan, DAI Qihang. Research progress of aqueous magnesium ion battery[J]. Acta Materiae Compositae Sinica, 2023, 40(7): 3756-3770 (in Chinese). [16] LI C, SHYAMSUNDER A, KEY B, et al. Stabilizing magnesium plating by a low-cost inorganic surface membrane for high-voltage and high-power Mg batteries[J]. Joule, 2023, 7(12): 2798-2813. doi: 10.1016/j.joule.2023.10.012 [17] NG K L, AMRITHRAJ B, AZIMI G. Nonaqueous rechargeable aluminum batteries[J]. Joule, 2022, 6(1): 134-70. doi: 10.1016/j.joule.2021.12.003 [18] RAMESH A, TRIPATHI A, BALAYA P. A mini review on cathode materials for sodium-ion batteries[J]. International Journal of Applied Ceramic Technology, 2022, 19(2): 913-923. doi: 10.1111/ijac.13920 [19] WANG G, WANG Z, SHI H, et al. Progress and perspective on rechargeable magnesium-ion batteries[J]. Science China Chemistry, 2022, 65: DOI doi: 10.1007/s11426-022-1454-0 [20] ELIA G A, KRAVCHYK K V, KOVALENKO M V, et al. An overview and prospective on Al and Al-ion battery technologies[J]. Journal of Power Sources, 2021, 481: 228870. doi: 10.1016/j.jpowsour.2020.228870 [21] WU F, YANG H, BAI Y, et al. Paving the path toward reliable cathode materials for aluminum-ion batteries[J]. Advanced Materials, 2019, 31(16): 1806510. doi: 10.1002/adma.201806510 [22] SHEN X, SUN T, WU Z, et al. Ultrafast charging and ultralong cycle life in solid-state Al-ion batteries[J]. Journal of Materials Chemistry A, 2022, 10(15): 8178-8185. doi: 10.1039/D2TA00630H [23] SHEN X, SUN T, YANG L, et al. Ultra-fast charging in aluminum-ion batteries: electric double layers on active anode[J]. Nature Communications, 2021, 12(1): 820. doi: 10.1038/s41467-021-21108-4 [24] CAI T, ZHAO L, HU H, et al. Stable CoSe2/carbon nanodice@reduced graphene oxide composites for high-performance rechargeable aluminum-ion batteries[J]. Energy & Environmental Science, 2018, 11(9): 2341-2317. [25] HUANG Z, SONG W-L, LIU Y, et al. Stable quasi-solid-state aluminum batteries[J]. Advanced Materials, 2022, 34(8): 2104557. doi: 10.1002/adma.202104557 [26] ZHOU L, YANG H, HAN T, et al. Carbon-based modification materials for lithium-ion battery cathodes: Advances and perspectives[J]. Frontiers in Chemistry, 2022, 10: 101446, doi: 10.3389/fchem.2022.914930 [27] HUANG H, ZHOU F, LU P, et al. Design and construction of few-layer graphene cathode for ultrafast and high-capacity aluminum-ion batteries[J]. Energy Storage Materials, 2020, 27: 396-404. doi: 10.1016/j.ensm.2020.02.011 [28] LIN M-C, GONG M, LU B, et al. An ultrafast rechargeable aluminium-ion battery[J]. Nature, 2015, 520(7547): 324-8. doi: 10.1038/nature14340 [29] WANG D-Y, WEI C-Y, LIN M-C, et al. Advanced rechargeable aluminium ion battery with a high-quality natural graphite cathode[J]. Nature Communications, 2017, 8(1): 14283. doi: 10.1038/ncomms14283 [30] LI Z, NIU B, LIU Y, et al. Prelithiation treatment of graphite as cathode material for rechargeable aluminum batteries[J]. Electrochimica Acta, 2018, 263: 68-75. doi: 10.1016/j.electacta.2017.12.166 [31] HU H, CAI T, BAI P, et al. Small graphite nanoflakes as an advanced cathode material for aluminum ion batteries[J]. Chemical Communications, 2020, 56(10): 1593-6. doi: 10.1039/C9CC06895C [32] DONG X, XU H, CHEN H, et al. Commercial expanded graphite as high-performance cathode for low-cost aluminum-ion battery[J]. Carbon, 2019, 148: 134-140. doi: 10.1016/j.carbon.2019.03.080 [33] DONG X, CHEN H, LAI H, et al. A graphitized expanded graphite cathode for aluminum-ion battery with excellent rate capability[J]. Journal of Energy Chemistry, 2022, 66: 38-44. doi: 10.1016/j.jechem.2021.07.016 [34] WANG J, TU J, LEI H, et al. The effect of graphitization degree of carbonaceous material on the electrochemical performance for aluminum-ion batteries[J]. RSC Advances, 2019, 9(67): 38990-38997. doi: 10.1039/C9RA07234A [35] ZHOU Z, LI N, WANG P, et al. All-carbon positive electrodes for stable aluminium batteries[J]. Journal of Energy Chemistry, 2020, 42: 17-26. doi: 10.1016/j.jechem.2019.03.027 [36] KONG Y, TANG C, HUANG X, et al. Thermal reductive perforation of graphene cathode for high-performance aluminum-ion batteries[J]. Advanced Functional Materials, 2021, 31(17): 2010569. doi: 10.1002/adfm.202010569 [37] RAJEEVAN S, JOHN S, GEORGE S C. The effect of poly(vinylidene fluoride) binder on the electrochemical performance of graphitic electrodes[J]. Journal of Energy Storage, 2021, 39: 102654. doi: 10.1016/j.est.2021.102654 [38] KUMAR C N S, KONRAD M, CHAKRAVADHANULA V S K, et al. Nanocrystalline graphene at high temperatures: insight into nanoscale processes[J]. Nanoscale Advances, 2019, 1(7): 2485-2494. doi: 10.1039/C9NA00055K [39] SONG Y, ZOU W, LU Q, et al. Graphene transfer: Paving the road for applications of chemical vapor deposition graphene[J]. Small, 2021, 17(48): 2007600. doi: 10.1002/smll.202007600 [40] HUANG H, ZHOU F, SHI X, et al. Graphene aerogel derived compact films for ultrafast and high-capacity aluminum ion batteries[J]. Energy Storage Materials, 2019, 23: 664-669. doi: 10.1016/j.ensm.2019.03.001 [41] SONI S K, THOMAS B, KAR V R. A comprehensive review on CNTs and CNT-reinforced composites: Syntheses, characteristics and applications[J]. Materials Today Communications, 2020, 25: 101546. doi: 10.1016/j.mtcomm.2020.101546 [42] ZHU S, YANG X, YAO Y, et al. One-dimensional heterostructures of polyoxometalate-encapsulated carbon nanotubes for enhanced capacitive energy storage[J]. Cell Reports Physical Science, 2023, 4(6): 101446. doi: 10.1016/j.xcrp.2023.101446 [43] QI S, SUN J, MA J, et al. Covalent bonding of sulfur nanoparticles to unzipped multiwalled carbon nanotubes for high-performance lithium–sulfur batteries[J]. Nanotechnology, 2019, 30(2): 024001. doi: 10.1088/1361-6528/aae6e5 [44] LIU S, CAO Z, MENG Y, et al. Aerophilic Co-embedded N-doped carbon nanotube arrays as highly efficient cathodes for aluminum–air batteries[J]. ACS Applied Materials & Interfaces, 2021, 13(23): 26853-26860. [45] ZHANG E, WANG J, WANG B, et al. Unzipped carbon nanotubes for aluminum battery[J]. Energy Storage Materials, 2019, 23: 72-78. doi: 10.1016/j.ensm.2019.05.030 [46] LIU Z, WANG J, DING H, et al. Carbon nanoscrolls for aluminum battery[J]. ACS Nano, 2018, 12(8): 8456-66. doi: 10.1021/acsnano.8b03961 [47] LI C, ZHANG X, HE W. Design and modification of cathode materials for high energy density aluminum-ion batteries: a review[J]. Journal of Materials Science:Materials in Electronics, 2018, 29(16): 14353-14370. doi: 10.1007/s10854-018-9478-1 [48] SOUNDHARRAJAN V, NITHIANANTH S, LEE J, et al. LiV3O8 as an intercalation-type cathode for aqueous aluminum-ion batteries[J]. Journal of Materials Chemistry A, 2022, 10(35): 18162-18169. doi: 10.1039/D2TA04823J [49] WANG S, JIAO S, WANG J, et al. High-performance aluminum-ion battery with CuS@C microsphere composite cathode[J]. ACS Nano, 2017, 11(1): 469-477. doi: 10.1021/acsnano.6b06446 [50] LI H, YANG H, SUN Z, et al. A highly reversible Co3S4 microsphere cathode material for aluminum-ion batteries[J]. Nano Energy, 2019, 56: 100-108. doi: 10.1016/j.nanoen.2018.11.045 [51] YIN X, REN Y, WU L, et al. Construction of polysulfides defense system for greatly improving the long cycle life of metal sulfide anodes for sodium-ion batteries[J]. Journal of Energy Chemistry, 2022, 71: 210-217. doi: 10.1016/j.jechem.2022.03.012 [52] AI Y, WU S-C, WANG K, et al. Three-dimensional molybdenum diselenide helical nanorod arrays for high-performance aluminum-ion batteries[J]. ACS Nano, 2020, 14(7): 8539-8550. doi: 10.1021/acsnano.0c02831 [53] SHADIKE Z, TAN S, WANG Q-C, et al. Review on organosulfur materials for rechargeable lithium batteries[J]. Materials Horizons, 2021, 8(2): 471-500. doi: 10.1039/D0MH01364A [54] LEE S, KWON G, KU K, et al. Recent progress in organic electrodes for Li and Na rechargeable batteries[J]. Advanced Materials, 2018, 30(42): 1704682. doi: 10.1002/adma.201704682 [55] TIE Z, NIU Z. Design Strategies for high-performance aqueous Zn/organic batteries[J]. Angewandte Chemie International Edition, 2020, 59(48): 21293-303. doi: 10.1002/anie.202008960 [56] YANG Z, WANG F, MENG P, et al. Recent advances in developing organic positive electrode materials for rechargeable aluminum-ion batteries[J]. Energy Storage Materials, 2022, 51: 63-79. doi: 10.1016/j.ensm.2022.06.018 [57] LU Y, CHEN J. Prospects of organic electrode materials for practical lithium batteries[J]. Nature Reviews Chemistry, 2020, 4(3): 127-142. doi: 10.1038/s41570-020-0160-9 [58] FRIEBE C, SCHUBERT U S. High-power-density organic radical batteries[J]. Topics in Current Chemistry, 2017, 375(1): 19. doi: 10.1007/s41061-017-0103-1 [59] BITENC J, LINDAHL N, VIZINTIN A, et al. Concept and electrochemical mechanism of an Al metal anode ‒ organic cathode battery[J]. Energy Storage Materials, 2020, 24: 379-383. doi: 10.1016/j.ensm.2019.07.033 [60] GUO F, HUANG Z, WANG M, et al. Active cyano groups to coordinate AlCl2+ cation for rechargeable aluminum batteries[J]. Energy Storage Materials, 2020, 33: 250-257. doi: 10.1016/j.ensm.2020.08.016 [61] YOO D-J, CHOI J W. Elucidating the extraordinary rate and cycling performance of phenanthrenequinone in aluminum-complex-ion batteries[J]. The Journal of Physical Chemistry Letters, 2020, 11(6): 2384-2392. doi: 10.1021/acs.jpclett.0c00324 [62] CASEY A, DIMITROV S D, SHAKYA-TULADHAR P, et al. Effect of systematically tuning conjugated donor polymer lowest unoccupied molecular orbital levels via cyano substitution on organic photovoltaic device performance[J]. Chemistry of Materials, 2016, 28(14): 5110-5120. doi: 10.1021/acs.chemmater.6b02030 [63] KIM D J, YOO D-J, OTLEY M T, et al. Rechargeable aluminium organic batteries[J]. Nature Energy, 2019, 4(1): 51-59. [64] LIU Y, LUO W, LU S, et al. Novel carbonyl cathode for green and sustainable aluminum organic batteries[J]. ACS Applied Materials & Interfaces, 2022, 14(48): 53702-53710. [65] HAN X, LI S, SONG W-L, et al. Stable high-capacity organic aluminum–porphyrin batteries[J]. Advanced Energy Materials, 2021, 11(32): 2101446. doi: 10.1002/aenm.202101446 [66] MAO M, LUO C, POLLARD T P, et al. A pyrazine-based polymer for fast-charge batteries[J]. Angewandte Chemie International Edition, 2019, 58(49): 17820-17826. doi: 10.1002/anie.201910916 [67] CHEN J, ZHU Q, JIANG L, et al. Rechargeable aqueous aluminum organic batteries[J]. Angewandte Chemie International Edition, 2021, 60(11): 5794-9. doi: 10.1002/anie.202011144 [68] LI H, FANG M, HOU Y, et al. Different effect of the additional electron-withdrawing cyano group in different conjugation bridge: The adjusted molecular energy levels and largely improved photovoltaic performance[J]. ACS Applied Materials & Interfaces, 2016, 8(19): 12134-12140. [69] CHEN H, XU H, WANG S, et al. Ultrafast all-climate aluminum-graphene battery with quarter-million cycle life[J]. Science Advances, 3(12): eaao7233. [70] HU Y, YE D, LUO B, et al. A binder-free and free-Standing cobalt sulfide@carbon nanotube cathode material for aluminum-ion batteries[J]. Advanced Materials, 2018, 30(2): 1703824. doi: 10.1002/adma.201703824 [71] ZHANG K, KIRLIKOVALI K O, LE Q V, et al. Extended metal–organic frameworks on diverse supports as electrode nanomaterials for electrochemical energy storage[J]. ACS Applied Nano Materials, 2020, 3(5): 3964-3990. doi: 10.1021/acsanm.0c00702 [72] WANG J, KIRLIKOVALI K O, KIM S Y, et al. Metal organic framework-based nanostructure materials: Applications for non-lithium ion battery electrodes[J]. CrystEngComm, 2022, 24(16): 2925-2947. doi: 10.1039/D1CE01737C [73] QIN T, QIN L, LI J. Metal–organic framework-derived CuTe@porous carbon composites as novel cathodes for aluminum ion batteries[J]. Ionics, 2022, 28(6): 2853-2863. doi: 10.1007/s11581-022-04530-6 [74] QIN L, QIN T, ZHONG J, et al. Electrochemical performance and Cu2+ modification of nickel metal organic framework derived tellurides for application in aluminum ion batteries[J]. Journal of Electroanalytical Chemistry, 2023, 928: 117014. doi: 10.1016/j.jelechem.2022.117014 [75] HUANG P, ZHANG S, YING H, et al. Fabrication of Fe nanocomplex pillared few-layered Ti3C2Tx MXene with enhanced rate performance for lithium-ion batteries[J]. Nano Research, 2021, 14(4): 1218-1227. doi: 10.1007/s12274-020-3221-y [76] LI Z, WANG X, ZHANG W, et al. Two-dimensional Ti3C2@CTAB-Se (MXene) composite cathode material for high-performance rechargeable aluminum batteries[J]. Chemical Engineering Journal, 2020, 398: 125679. doi: 10.1016/j.cej.2020.125679 [77] KLIMPEL M, KOVALENKO M V, KRAVCHYK K V. Advances and challenges of aluminum–sulfur batteries[J]. Communications Chemistry, 2022, 5(1): 77. doi: 10.1038/s42004-022-00693-5 [78] YANG H, YIN L, LIANG J, et al. An aluminum–sulfur battery with a fast kinetic response[J]. Angewandte Chemie International Edition, 2018, 57(7): 1898-1902. doi: 10.1002/anie.201711328 [79] ZHANG X, JIAO S, TU J, et al. Rechargeable ultrahigh-capacity tellurium–aluminum batteries[J]. Energy & Environmental Science, 2019, 12(6): 1918-27. [80] ZHANG X, TU J, WANG M, et al. A strategy for massively suppressing the shuttle effect in rechargeable Al–Te batteries[J]. Inorganic Chemistry Frontiers, 2020, 7(20): 4000-4009. doi: 10.1039/D0QI00841A [81] LI Z, LV W, WU G, et al. Hollow nanotubes carbon@tellurium for high-performance Al-Te batteries[J]. Electrochimica Acta, 2022, 401: 139498. doi: 10.1016/j.electacta.2021.139498 [82] ZHANG X, WANG M, TU J, et al. Hierarchical N-doped porous carbon hosts for stabilizing tellurium in promoting Al-Te batteries[J]. Journal of Energy Chemistry, 2021, 57: 3783-85. -
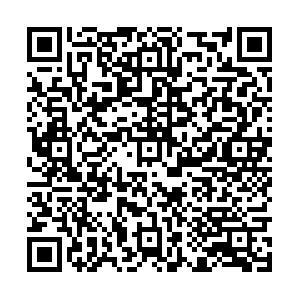
计量
- 文章访问数: 135
- HTML全文浏览量: 73
- 被引次数: 0